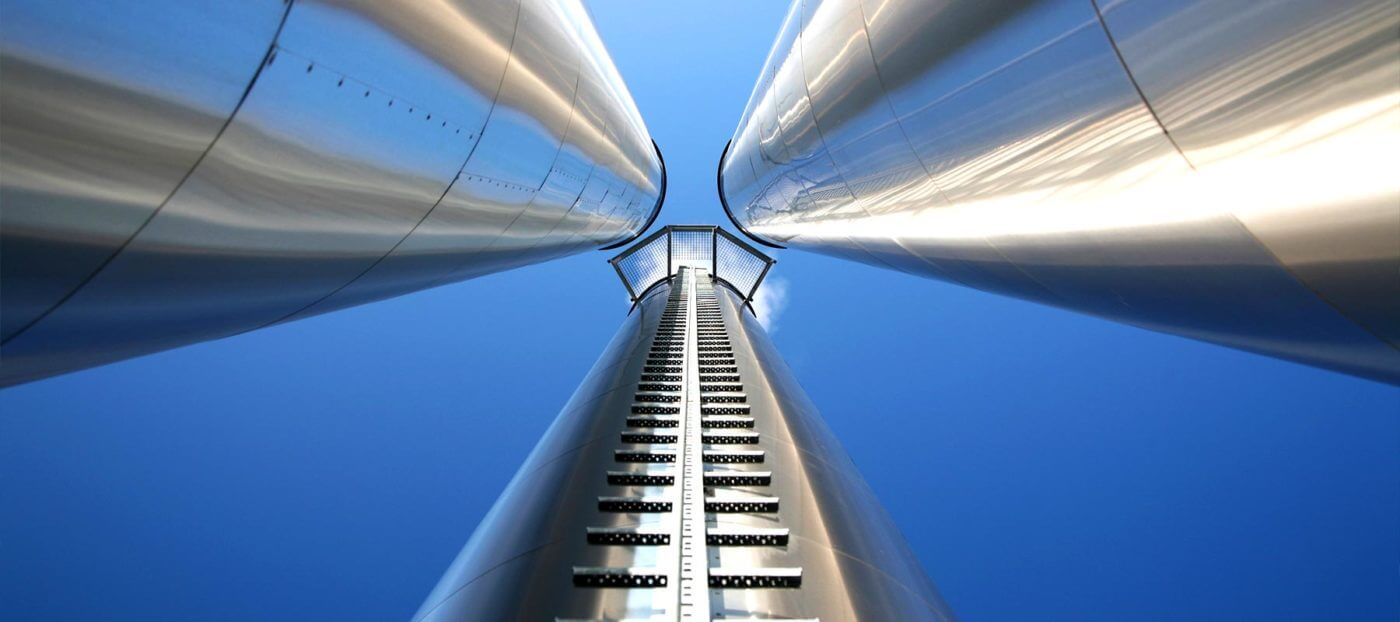
DAC-LAT: A new tool for navigating the complexities of direct air capture
As the world grapples with climate change, the concept of achieving net zero emissions by 2050 and ‘net negative’ thereafter has emerged as a cornerstone of climate policy. Central to this goal is using carbon dioxide removal (CDR) to balance residual emissions by removing an equivalent amount of CO2 from the atmosphere. While there is no one existing definition for residual or ‘hard-to-abate’ emissions, we can think of them as emissions that are expected to be very technically challenging or economically unfeasible to mitigate at source. Sectors such as aviation, agriculture, or the remaining emissions leftover after applying carbon capture and storage (CCS) to cement production come to mind. Another use of carbon removals is to deliver ‘net negative’ emissions, another goal of the EU post ‘net zero’ whereby more carbon is removed from the atmosphere than is added.
One such set of technologies to deliver carbon removals is direct air capture (DAC). DAC extracts CO2 directly from ambient air using chemical processes. Projects primarily employ either liquid solvents or solid sorbents to capture CO2, which is then compressed and transported to storage or utilized. When paired with permanent storage methods like geological sequestration or mineral carbonation, DAC becomes a carbon dioxide removal solution known as DACS.
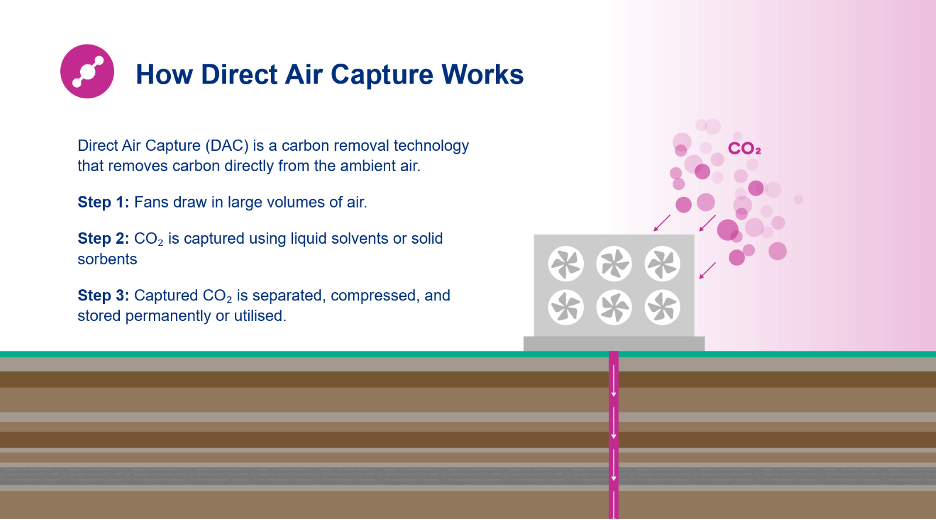
How does DAC capture CO2?
There are many different variants of DAC technology under development, but there are two leading technology types that are currently being deployed at relatively large scales (capturing tens of thousands of tonnes of CO2 annually or more):
- Solid Sorbent DAC (S-DAC): Air is passed over solid materials that chemically bind with CO2. The sorbent is then heated to a temperature below 100ºC to release the captured CO2 for storage.
- Liquid Solvent DAC (L-DAC): Air is exposed to a liquid solution that absorbs CO2 and chemically converts it to solid carbonate pellets. A high temperature kiln (~900ºC) is then used to break down the carbonate and release pure CO2.
While DAC is energy-intensive, its operational advantages – such as flexibility, scalability, and modularity – make it a promising tool for carbon removal. Moreover, the ability to verify and monitor negative emissions with precision adds to its appeal.
However, not all countries have the available resources to make efficient use of DACS, as the process can be highly energy intensive. This means that consideration of the greenhouse gas intensity of its energy inputs is vital to determine its ability to deliver net removals. That’s where our new Direct Air Capture Lifecycle Analysis Tool (DAC-LAT) comes in.
The Direct Air Capture Lifecycle Analysis Tool
What is DAC-LAT?
DAC‐LAT is an interactive online tool that lets you estimate the net CO₂ removal achieved by a DAC facility. Here’s how it works:
- Interactive input selection: Users begin by choosing the Global Warming Potential (GWP) time horizon and selecting which DAC technology to evaluate. The tool currently allows the user to choose between the two archetypal DAC methods based on solid sorbents and liquid solvents
- Customizable parameters: Next, you can set the greenhouse gas intensities of electricity and heat used by the facility, based on national and regional electricity grids and natural gas supply chains, a single, dedicated renewable or fossil energy source, and even future projections of grid carbon intensity. The user can also set the plant’s operational lifetime and the fraction of time the facility is active over a year. In addition, while the tool doesn’t provide a full capital cost analysis, it does allow you to estimate the cost of energy inputs by entering unit prices for electricity and heat.
Comprehensive emissions breakdown
DAC‐LAT calculates emissions from each stage of the DAC value chain – from the energy consumed during capture and the construction of the facility to the CO₂ compression, transport, and storage processes. This holistic approach reflects recent findings that emphasize the importance of including all indirect emissions to determine true net removal. We hope that developers of CDR standards take note, as accurate certification schemes and standards should aim to lay a foundation of trust, and such a foundation can only be built by providing accuracy.
Case study: Liquid-DAC in California vs. Solid-DAC in France
To illustrate how the Direct Air Capture Lifecycle Analysis Tool (DAC-LAT) can support decision-making, let’s examine two scenarios that highlight the impact of different technology choices, energy inputs, and regional settings on net CO₂ removal.
Case 1: Liquid Sorbent DAC in California (U.S. grids, natural gas with recapture)
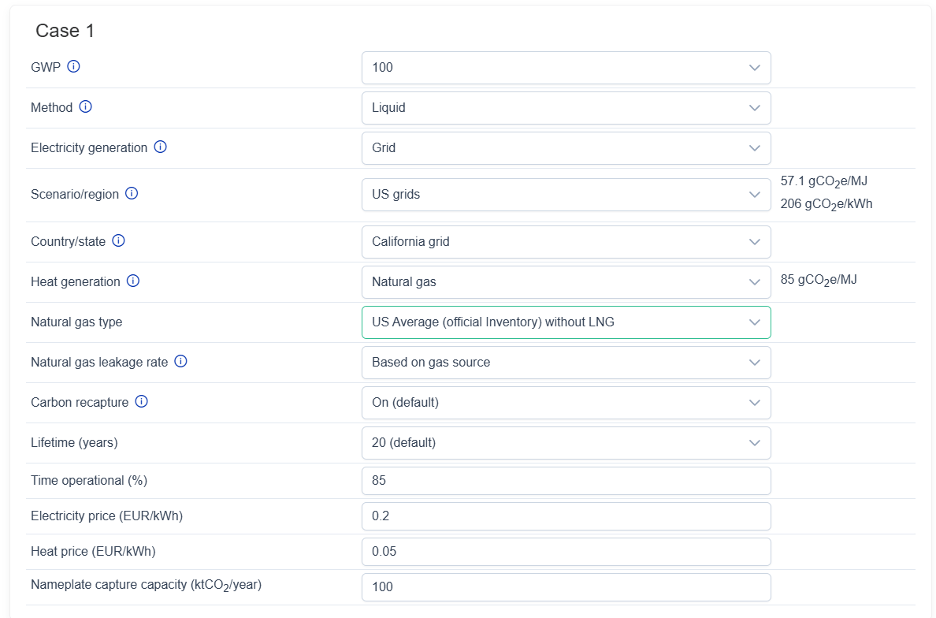
In this scenario, a liquid-based DAC facility operates in California, using grid electricity and heat from natural gas with carbon recapture. This means that CO2 produced by the natural gas in the high-temperature sorbent regeneration process is also captured and stored along with the atmospheric CO2. The plant has a capture capacity of 100 ktCO₂/year and is designed to run 85% of the time over a 20-year lifetime.
Key findings
Energy Inputs | |
---|---|
Electricity | 1.23 MJ/kg CO₂ |
Heat | 6.28 MJ/kg CO₂ |
Total Lifecycle Emissions | 383.2 gCO₂e per kg CO₂ stored |
Largest Emission Sources | Heat (244.7 gCO2e per kg CO2), electricity (70.2), and chemicals (21.34) |
Total CO₂ Stored | 109.57 ktCO₂/year, of which 85 ktCO2/year is atmospheric CO2 |
This case illustrates the emissions burden associated with fossil fuel-based heat, even with recapture, and the significant impact of energy inputs on the total carbon balance. CO2 and methane emissions from the natural gas supply chain contribute over 30% of the total lifecycle emissions. Other uncaptured emissions associated with natural gas combustion for heat could potentially be abated using lower-carbon sources of heat.
Case 2: Solid Sorbent DAC in France (with grid electricity and heat from nuclear power)
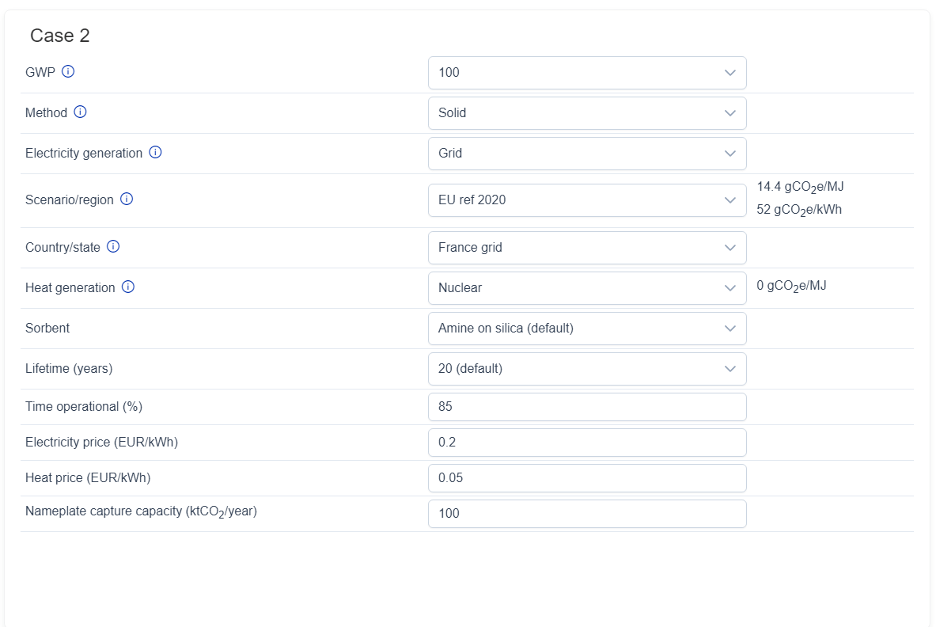
A second scenario models a solid-based DAC facility located in France, leveraging France’s low-carbon grid electricity and drawing heat from the steam cycle of a nuclear power plant. For the solid process, the user can also choose from several different sorbents, and the basic case of silica-supported amines is selected here. The plant retains the same capture capacity (100 ktCO₂/year) and operational parameters as in Case 1.
Key findings
Energy Inputs | |
---|---|
Electricity | 2.52 MJ/kg CO₂ |
Heat | 11.9 MJ/kg CO₂ |
Total Lifecycle Emissions | 79.66 gCO₂e per kg CO₂ stored |
Largest Emission Sources | Chemicals (24), electricity (36.22), and storage (6.11) |
Total CO₂ Stored | 85 ktCO₂/year |
This scenario highlights the benefit of low-carbon electricity sources, such as nuclear energy, in minimizing lifecycle emissions. The emissions are nearly four times lower than in Case 1, underscoring how energy sourcing significantly influences DAC’s climate impact.

DAC‐LAT is designed to help you see the bigger picture: that while a DAC facility can remove carbon from the atmosphere, there can be stark differences between just how effective a facility will be at delivering ‘net removals’. With limited time and resources, it is paramount to make an effective use of both. Deciding what DAC technology to choose and where to place the facility are crucial, and we hope this tool can inform those choices by highlighting promising countries and regions for deploying DAC as well as the importance of comprehensive lifecycle analysis when designing standards.