Soluciones para una transición neta cero en el Reino Unido
Recommendations for UK policy
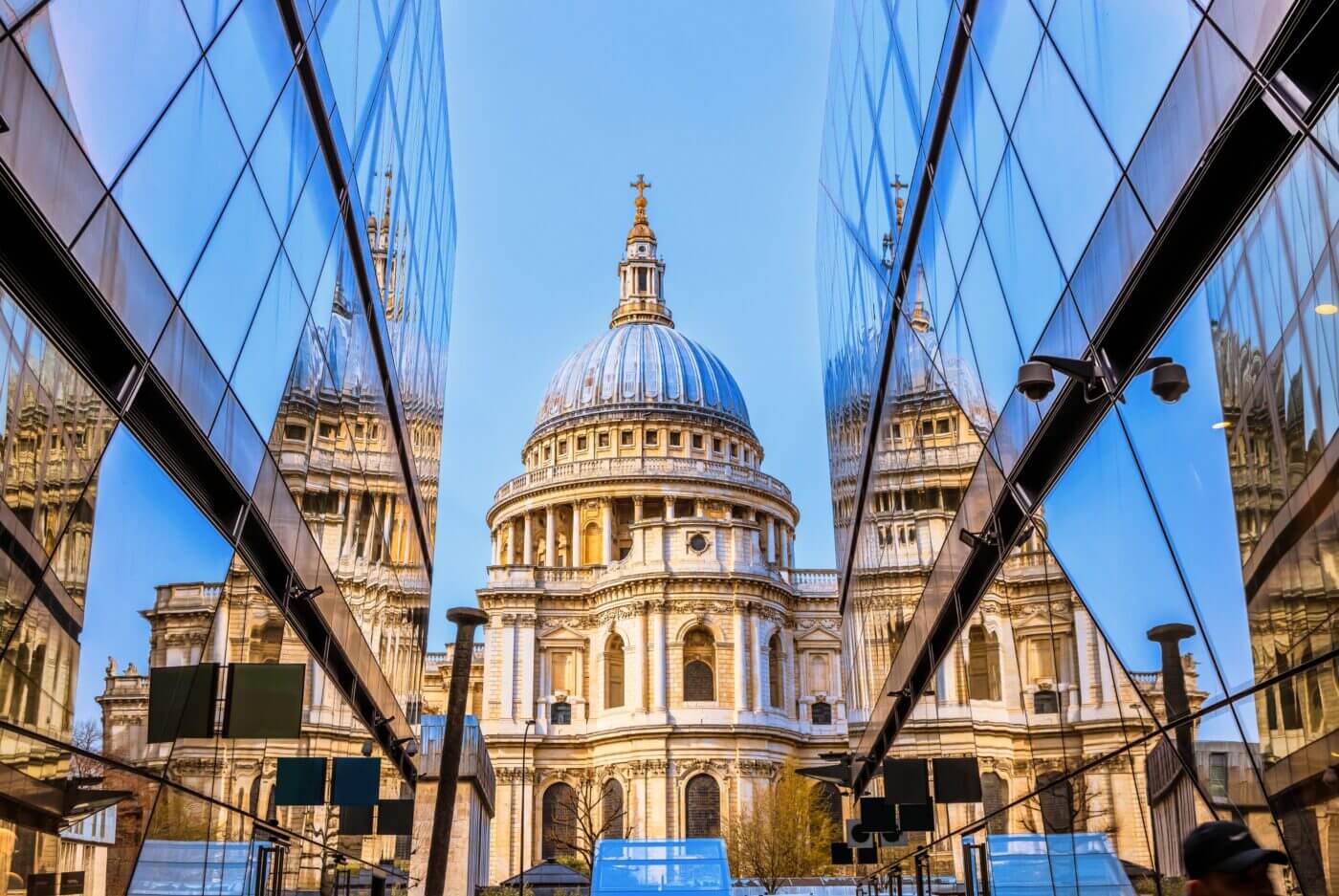
Acerca de este informe
This report outlines key recommendations to reinvigorate the UK’s climate leadership in this crucial decade of the transition. It emphasises the need to focus on real deployment of climate and energy policy, as well as to forge partnerships that will help to facilitate both domestic and international implementation. It offers a solutions-based approach, highlighting the key roles that methane mitigation, carbon capture, removal and storage, low-carbon hydrogen, transport decarbonisation and nuclear will have to play in enabling the UK to decarbonise its energy system and meet its climate targets.
Autor del informe
Rebecca Tremain, Head of UK Government Affairs, CATF
Colaboradores principales
Alex Carr, Program Manager, Zero-Carbon Fuels, CATF
Sehila Gonzalez, Global Director, Fusion Energy, CATF
Brandon Locke, Director de Política Europea, Prevención de la Contaminación por Metano, CATF
Toby Lockwood, Technology and Markets Director, Carbon Capture, CATF
Lily Odarno, Director, Energy and Climate Innovation, Africa,CATF
Malwina Qvist, analista principal, CATF
Codie Rossi, Policy Associate, Carbon Capture, CATF
Kasparas Spokas, Director de Perspectivas y Estrategia de Integración, CATF
John Steelman, Deputy Director, Transportation Decarbonization, CATF
James Turrito, Director, Global Campaigns, Methane Pollution Prevention,CATF
Alessia Virone, Government Affairs Director, Europe, CATF
Gus Whakim, Production and Export Director, Zero-Carbon Fuels, CATF
Overview
The UK has long been regarded as a climate leader and has achieved clear progress, cutting its greenhouse gas emissions by 50% since 1990. There are important things to learn from the UK’s experience — it was the first major economy to legislate for net zero and the first to set legally binding carbon budgets. The UK also has a successful history of policy innovation, modelling new approaches to policy and legislation that have subsequently become widely adopted practices, such as the Contracts for Difference (CfD) scheme. Furthermore, as former COP26 Presidency, the UK spent over three years leading international climate diplomacy under the auspices of the UNFCCC. The UK’s extensive diplomatic experience and reach provide an opportunity to further its global partnerships and share its experience internationally.
However, the most recent Climate Change Committee (CCC) Progress Report concluded that the UK is off track to meet both its 2030 nationally determined contribution (NDC) and its legally-binding sixth carbon budget (2033-2037). The Russia-Ukraine war and resulting impacts on energy prices, supply chains, and the cost-of-living have stalled ambition and progress on implementation. This has also led to Government row backs on existing net zero policies, putting the UK’s targets and leadership position even further in jeopardy. And there are limits to the progress that can be made using existing policy instruments. The UK is therefore at risk of ceding its global leadership position on climate, and with it its ability to seriously compete in the industries of the future.
We are now in the crucial decade in the fight against climate change. Climate remains a top concern amongst voters; there is widespread public support in the UK for a range of clean firm energy sources, including nuclear, carbon, capture and storage (CCS) and low-carbon hydrogen. Moreover, the world’s largest economies such as the US and China, are planning to invest heavily in clean technologies. Clean technology has the potential to support UK energy security in a time of geopolitical complexity, to drive long-term competitiveness for the UK economy, and to facilitate implementation of the UK’s climate goals.
The upcoming General Election provides an opportunity for the UK to secure climate breakthroughs at the national level, even if the long-run impacts of previous crises continue to complicate the picture. The focus for the UK’s re-commitment to climate policy must be on accountability and implementation, with a focus on delivery and real deployment of climate and energy policy. CATF is therefore focusing on:
- Supporting the deployment of realistic and systems-centred solutions that will enable the UK to match its world-leading ambition with world-leading delivery, through policy innovation and development in
- Methane mitigation, particularly in the oil and gas sector;
- Carbon capture, removal, and storage;
- Low-carbon hydrogen in no-regrets sectors;
- Transport decarbonisation; and
- Nuclear.
- Sharing learnings from the UK’s experience to support decarbonisation in other regions including Europe, MENA, the US and Africa.
- Developing global solutions to tackling climate change, such as a playbook to deploy nuclear energy at scale, and supporting the creation of a global fusion industry.
- Facilitating collaboration on climate action between the UK and other key partners, including with the EU and its Member States.
Challenges and obstacles to decarbonisation
As articulated by the International Energy Agency (IEA) in its Net Zero by 2050 report, “achieving net zero emissions by 2050 will require nothing short of the complete transformation of the global energy system.” It is widely understood that part of this transformation requires the immediate deployment of existing commercial zero-carbon technologies like solar and wind electricity, along with the electrification of large parts of the buildings, industry and transportation sectors. However, electrification alone cannot address the climate challenge.
The UK recognises the need to deploy the complete set of available decarbonisation technologies, including low-carbon hydrogen, carbon capture and storage and nuclear, and recognises the importance of an economy-wide, systems-based approach to the energy transition. A diverse technology strategy helps to reduce the costs of to-be-developed technologies in the international community, helping to meet energy access, energy security and energy reliability needs; eliminate energy poverty; and foster economic growth in low-income countries.
The UK also acknowledges several key challenges in meeting its climate targets, including difficult to electrify sectors such as heavy industry, heavy-duty transport, high temperature industrial process heating and long-haul aviation – where electrification is not currently technically or economically feasible and will require alternative solutions; the need for a strong market demand for emerging technologies; the need to mitigate against carbon leakage ensuring domestic industry can transform quickly and efficiently; and the need for new market mechanisms to support the transition to a low-carbon economy (e.g. support and incentives for CCS).
However, additional challenges to decarbonisation should be addressed to improve the likelihood of a successful transition. Several obstacles pose a threat to the pace and scale of decarbonisation in the UK:
- Transition planning: A rigid adherence to specific forecasts and an overconfident narrative perpetuated across the energy industry has led to unexpected outcomes such as failed procurements and cancellations of offshore wind in the UK. Thus, UK policymakers must remain open to many plausible futures that will evolve dynamically. Recent macro-economic and geopolitical events have shown that the costs of technologies, capital, and the availability of supply chains are all subject to significant uncertainty. A timely and periodic review of crucial technologies, along with their costs and associated uncertainties, will be necessary. Moreover, UK officials should conduct risk assessments at the portfolio level to clearly identify shortcomings in chosen decarbonisation pathways across all elements. This will enable targeted actions to safeguard against the failure to meet climate and economic targets.
- Land availability limitations: Intermittent renewables have a large land use footprint, which may become challenging with competing land uses (e.g., urbanisation, agriculture, carbon sequestration and conservation values) as more are needed to meet existing and incremental electrification demand (Figure 1). Decreasing land availability can limit the pace of renewables development both from a logistical siting standpoint, but also increase building costs as the price of land increases (Figure 2). This obstacle can be mitigated by maximising the use of high energy density electricity sources, such as nuclear and hydrogen. Both technologies are supported by the UK Government, however, their positive impact on land availability should be evaluated and accounted for.
- Variability (daily, seasonal, and annual): Solar and wind are valuable energy resources but have seasonal variation in output over timespans that are longer than can be managed with current battery storage technologies. The UK’s ambitious target for 50 GW of offshore wind by 2030 will increase the variability and volatility of the electricity supply. While the UK’s Net Zero Strategy calls for demand side flexibility by shifting electric vehicle (EV) charging, the use of smart meters, and shifting heating windows, there is little empirical evidence suggesting large-scale demand management that can address weekly and seasonal variability is feasible either operationally or socially. Maintaining adequate amounts of zero-carbon dispatchable power in the electricity system, alongside long-duration energy storage, can help to mitigate this challenge.
Figure 1: Land intensity of two projected electricity system decarbonisation (55% of total final energy demand) scenario results for the UK [Source: Carbon Free Europe modelling]
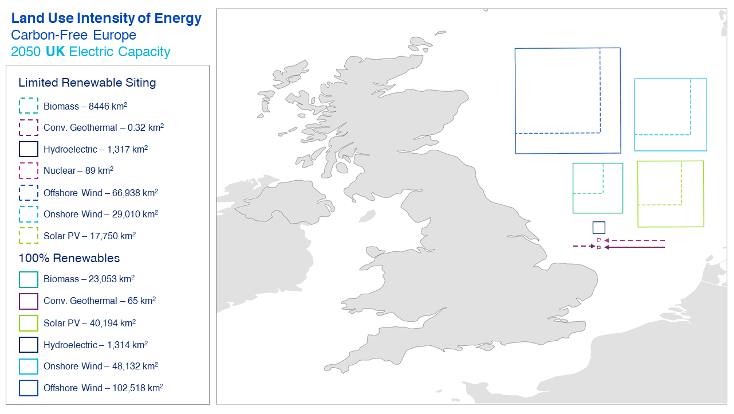
- Electricity transmission bottlenecks: The UK seeks to address transmission bottlenecks through its transmission acceleration action plan, recognising the criticality of transmission growth as a lynchpin of the energy transition. A part of the solution already being explored by Ofgem can be introducing locational price signals that would foster siting new generation projects nearer demand centres. As electricity demand increases due to electrification, we will need to reconductor transmission lines to increase capacity while also building new trunk lines. Simultaneously, expansion of variable renewable technologies will require an unprecedented number of spur lines to be developed. Without adequate proactive commitments for grid tie-ins, variable renewable developers are unlikely to develop projects at the pace and scale necessary to meet the UK’s climate goals.
- Supply chain limitations: Economy-wide decarbonisation across the globe will pressure already strained supply chains to deliver large scale infrastructure at an unprecedented pace and scale in the next couple of decades. Supply of critical minerals and their supply chains may limit the speed and deployment of solar, wind, and battery capacity. Additionally, the location of critical minerals and other supply chain inputs may be vulnerable to geopolitical disruptions. Even technologies that do not rely on critical minerals may face supply chain limitations. Due to the nature of its supply chains, the nuclear industry can tend to be oligopolistic, which can lead to backlogs and prolonged waiting times for nuclear plant components. Similar conditions apply to the renewable sector where multiple countries will compete for capital equipment and workforce on a similar timescale.
- Undeveloped low-carbon hydrogen and carbon storage infrastructure: The large-scale adoption of low-carbon hydrogen and CCS requires new pipeline and storage infrastructure to be deployed at unprecedented rates. They also require relatively high capital costs, a high level of coordination, long-term planning for infrastructure, and a trade-off between maximising inter-business and inter-regional cooperation and knowledge sharing while moving towards a competitive market.
- Lagging planning, permitting, and social acceptance: Around the world, there is a lack of proactive planning to facilitate building carbon-free energy infrastructure and increasing social pushback (Figure 2). In the UK, projects face wait times of up to 15 years to connect to the grid. This means that, even in sectors where electrification is viable to meet climate goals, a non-negligible risk of failure exists due to potential pushbacks and delays.
Figure 2: Schematic visualising how the amount of renewable deployment (y-axis) changes as more projects are developed (x-axis)
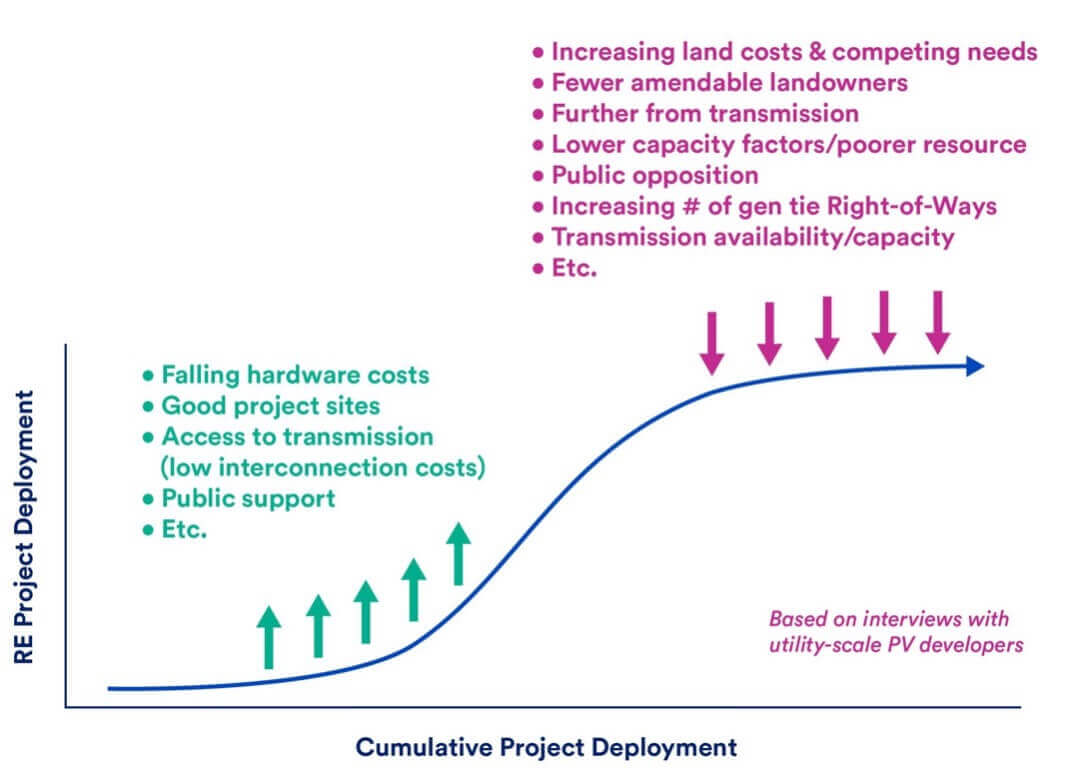
- Continuing non-CO2 emissions: Methane is a powerful super pollutant that is more than 80 times as powerful as carbon dioxide in warming our planet over a 20-year period. The report of the Sixth Working Group of the Intergovernmental Panel on Climate Change (IPCC), found that methane is already responsible for 0.5°C of total net warming (1.1°C) over the last decade. As such, this greenhouse gas must play a critical role in the UK’s decarbonisation strategy. Without fast, comprehensive action to reduce methane pollution, any long-term decarbonisation strategy will fail to meet climate targets.
- Unintended policy outcomes: The UK government must ensure that low-carbon electricity used to meet low-carbon hydrogen (and other low-carbon fuels) production goals is additional, and results in a decrease in overall carbon emissions across the economy, rather than shifting the emissions to sectors which are currently exempt from carbon reporting, or overseas where carbon accounting policies are not as developed.
- Market sequencing obstacles — “chicken-and-egg” problems: As already highlighted in some of the examples above, many choices made by developers and consumers depend on other market conditions, which in turn rely on the choices made by developers and consumers. As an example, investors in carbon capture facilities will be hesitant to make final investment decisions if carbon dioxide transport infrastructure (i.e., pipelines) are not readily available. However, investors in transport infrastructure will also be hesitant to develop pipelines without contracts to transport captured carbon. Such situations exemplify the key role for governments in the transition to support local industries. To achieve targets and catalyse economic growth, it will be key for governments to proactively plan, invest, and develop socially shared infrastructure in partnership with private industry to enable their subsequent development and emissions reductions, as well as job creation.
- Incomplete life cycle emissions accounting: The UK’s carbon accounting system should take into consideration the full life cycle emissions generated by technologies necessary to reach the net zero goal, including renewable technologies. Some of the solutions currently included in the UK’s Net Zero Strategy contain moderate upstream emissions (generated prior to deployment in the UK, these emissions can be embedded in the materials used to produce the capital equipment or released during the production process, e.g., electricity used in economies with high-grid carbon intensity) and are associated with other issues such as air quality. These emissions should be taken into consideration when making policy decisions around technology deployment and supply chain requirements. For example, energy from imported woody biomass should be further scrutinised for embedded emissions due to the long-distance transport and processing of this feedstock as well as resourcing sustainability concerns.
Tackling methane emissions
Tackling methane emissions from the oil and gas sector is one of the fastest, most cost effective, and impactful actions the UK can take to address the energy, cost-of-living, and climate crises. Whilst the UK has made good progress tackling methane emissions from the energy sector, this has mostly been as a co-benefit of the closure of coal plants. The UK hosted and facilitated the launch of the Global Methane Pledge at COP26, which aims to reduce global methane emissions by 30% by 2030. It remains one of the signature climate initiatives from the UK’s tenure as COP Presidency. As such, the UK has an interest in seeing progress towards Pledge commitments. At COP28, the UK committed $2.5 million to the Methane Finance Sprint, where funds will support cutting methane emissions across all sectors with a focus in low- and middle-income countries.
The UK must continue to build on the actions set out in its methane memorandum and develop comprehensive methane mitigation policies in addition to supporting international efforts to reduce emissions globally.
Key Policy Recommendations
- Establish a robust Methane Action Plan that outlines sectoral objectives and targets to reduce methane emissions, along with corresponding policies and measures.
- Bring forward a ban on routine venting and flaring and put this into law.
- Require quarterly leak detection and repair (LDAR) inspections by oil and gas installations to reduce fugitive methane emissions, together with a measurement-based monitoring, reporting and verification process to quantify methane emissions.
- Introduce a methane fee based on emissions intensity of oil and gas produced domestically (similar to that of the US).
- Implement a methane border adjustment mechanism on all oil and gas imported into the UK (aligning with EU plans for a Methane Import Standard).
- Capitalise on the UK’s role as COP Presidency during the announcement of the Global Methane Pledge and increase international engagement in and public financing of programmes (like the Climate and Clean Air Coalition and the International Methane Emissions Observatory) to reduce methane emissions.
Background
¿Por qué las emisiones de metano?
Climate benefits: Methane is the second greatest contributor to climate change and over 80 times more potent than CO2 for global warming over a period of 20 years. Methane mitigation is one of the most cost-efficient methods to have a fast impact on the global warming process, slow down global warming, and avoid irreversible tipping points. It is also one of the only low-hanging fruits remaining in the climate fight, a measure that has very limited to no cost and can have a major beneficial impact for climate. The Sixth IPCC Assessment Report identified methane mitigation as a priority and stressed the need for rapidly reducing methane emissions.
Energy security benefits: In the context of the energy crisis, cutting methane emissions would ensure that all the gas in the pipeline arrives to consumers. North Sea oil companies are currently wasting enough gas to supply 760,000 average UK homes each year. A study by Princeton also found that the UK is severely underestimating its methane emissions, with as much as five times more methane being leaked from oil and gas production than reported.
Economic benefits: As the price of energy rises, cutting methane emissions has become more economically beneficial. Venting and flaring methane during oil and gas operations is a major economic loss. The International Energy Agency estimates that globally, nearly $60 billion was lost due to venting and flaring in 2021. During the last decade, the UK has wasted £2.6 billion in lost gas sales due to flaring and venting and released 45 million tonnes of carbon dioxide into the atmosphere. The IEA has shown that oil and gas operators in the UK can waste 72% less methane by tackling methane leaks, venting and flaring, with existing technologies, much of which would be profitable actions for companies.
International context: Methane emerged as a global climate priority at COP26, where the British government supported the creation of the Global Methane Pledge, endorsed by over 150 countries around the world. Signatories committed to collectively reduce global methane emissions by at least 30% by 2030, from a 2020 baseline. UK partners are moving forward with ambitious measures: Canada is planning monthly LDAR at all oil and gas sites; the US adopted a methane tax fee and will soon come up with LDAR rules; and the EU agreed its first ever rules on reducing methane emissions in the energy sector. In 2021, the UK produced roughly half as much oil as Norway yet flared 5 times as much natural gas. The UK risks lagging behind its partners, with rules less ambitious than the rest of the world. The UK should develop a robust Methane Action Plan within the framework of the Global Methane Pledge, which establishes clear objectives across the energy, waste, and agriculture sectors, with detailed timelines and benchmarks for execution.
UK Methane Border Adjustment Mechanism: As an importer and exporter of oil and gas, the UK is in a unique position to reap significant economic benefits from a methane fee that could target energy produced both domestically and abroad. According to the IEA, the UK’s oil and gas sector emitted over 222kt of methane in 2022, which corresponds to £2.4 million1 (based on gas prices in the UK in October 2023) lost to the atmosphere. Implementing a methane fee and border adjustment mechanism would therefore help incentivise necessary emissions reductions, while bringing in valuable government funding. Furthermore, roughly 58% of the UK’s oil exports go to the EU, which will be subject to the forthcoming import standard in the EU Methane Regulation, and associated rules on MRV and methane intensity. Therefore, implementing a methane fee would help ensure the UK’s exports remain competitive on the EU market.
United States Waste Emissions Charge (aka “The Methane Fee”)
The Methane Emissions Reduction Program (MERP) included in the Inflation Reduction Act establishes a charge on gas wasted to the atmosphere and creates an incentive for operators to reduce their methane emissions. MERP will incentivise operators to reduce emissions sooner rather than wait until Environmental Protection Agency (EPA) rules are required to be fully implemented in five years. The MERP requires EPA to impose a charge on oil and gas sources (the Waste Emissions Charge) that are already required to report to EPA’s Greenhouse Gas Reporting Program (GHGRP), for any emissions those sources report above a segment-specific threshold set by the bill:
- Production: 0.20 percent of gas sent to sale or 10 metric tons of methane per million bbl oil sent to sale
- Processing/Gathering and Boosting/LNG: 0.05 percent
- Transmission and Storage: 0.11 percent
The fee begins at $900/ton in 2024, before increasing to $1,200/ton in 2025 and settling at $1,500/ton in 2026. As recently proposed, once EPA has made a determination that plans to implement existing source standards are in place and achieve the required methane reductions – a process that could take 3 years or more – sources in full compliance with the EPA rules will then be exempt from the charge. Emissions caused by a lengthy delay in permit approvals for infrastructure needed to remove gas from a facility can also be exempted.
EU Methane Regulation
In November 2023, the EU agreed its first ever rules on reducing methane emissions in the energy sector. The regulation introduces new requirements for the oil, gas and coal sectors to measure, report and verify methane emissions, and put in place mitigation measures to avoid such emissions, including detecting and repairing methane leaks and limiting venting and flaring. It also puts forward global monitoring tools to ensure transparency on methane emissions from imports of oil, gas and coal into the EU.
Methane Import Standard
The regulation includes landmark obligations on importers of fossil fuels. These obligations will be implemented in a phased approach, with data and reporting obligations starting first, just nine months after the Regulation’s entry into force.
From 2027, importers will be required to demonstrate that they meet the same MRV standards as those adopted in the EU’s methane regulation. The Commission will set forth a methodology for a methane intensity standard, which will be adopted by 2030. CATF’s analysis with Rystad showed that a phased import standard would have demonstrable emissions reduction benefits, with few negative impacts on EU energy security and the price of oil and gas. This is due to the evolving oil and gas market and the low marginal costs for compliance, combined with the increased potential for expansion of clean energy resources.
Con la previsión de que los nuevos suministros de energía modifiquen drásticamente los mercados mundiales a partir de 2025, se espera que la norma de importación tenga un coste mínimo para los proveedores de gas, y un impacto aún menor en los consumidores, porque muchos proveedores podrán reducir suficientemente las emisiones para evitar el pago de una tasa - dejando a los que sí tengan que pagar la tasa con poco poder de fijación de precios para repercutir la tasa en los consumidores. En el modelo de CATF y Rystad, los precios subirán un 1% - como mucho - debido a la norma de importación.
Figure 3: Crude oil exports and methane intensity of top export countries to the EU, 2023
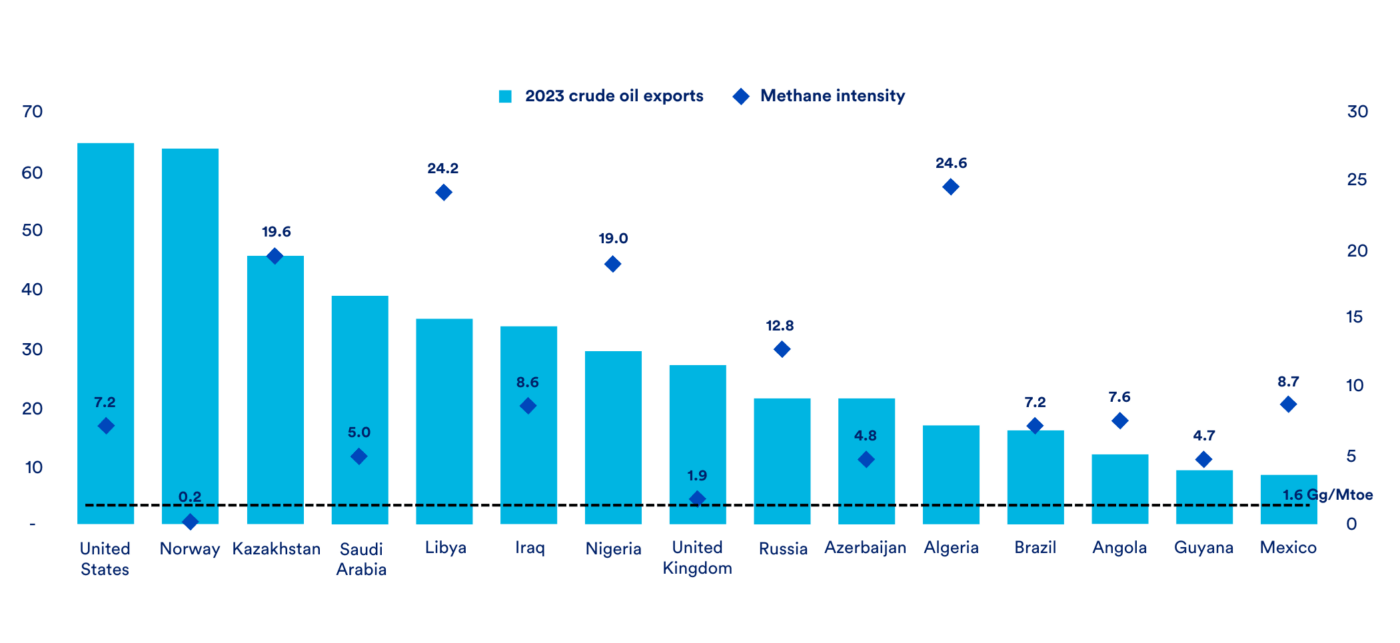
Descarbonización industrial
Climate policies have recently encountered backlash and raised concerns from industries, policymakers, and citizens, in the context of the energy crisis, rising living prices, and industrial competitiveness concerns. There has been a trend towards industrial de-specialisation globally, as countries attempt to compete in every stage of technology value chains, rather than just those in which they have a comparative advantage. The COVID-19 pandemic’s disruption of global supply chains, the rise of new competitors, and protectionism have all contributed to new industrial strategies that prioritise domestic control.
In response, there has been a wave of new industrial policies across regions — including the Inflation Reduction Act (IRA) in the United States, the Net Zero Industry Act (NZIA) in the European Union, major new investments into solar and hydrogen production in India, and increasing industrial investment across regions, that intend to localise or in some cases re-shore clean energy production. In the UK, the Chancellor announced in the Autumn Statement funding of £4.5 billion to attract investment to strategic manufacturing sectors, with £960 million earmarked for clean energy through a Green Industries Growth Accelerator, including carbon capture, utilisation and storage, electricity networks, hydrogen, nuclear and offshore wind.
Industry is the UK’s third highest-emitting sector, responsible for 14% of UK emissions in 2022. For the UK to hit its climate targets, the pace of industrial decarbonisation needs to speed up to around 8% per year out to 2030 (industry emissions fell by 3% in 2022). Whilst the UK will not be able to compete at the scale of the IRA or the NZIA (the UK’s market size is five times smaller than the EU and seven times smaller than the US), and it should not attempt to compete in every stage of technology value chains, Government should further reinvigorate the UK’s industrial strategy in areas of comparative advantage to meet the UK’s growth and decarbonisation objectives.
Key Policy Recommendations
- Government should develop a clear plan for industrial decarbonisation through the use of key technologies such as electrification, hydrogen, and CCS.
- Create a clear decision-making and coordination body within Government to facilitate a joined-up, targeted and strategic approach to industrial decarbonisation.
- When making decisions about potential technologies, policymakers should assess solutions against key criteria, including site specific (e.g., land availability, grid connection, clustering and energy constraints), technical (e.g., process reliability, flexibility and efficiency, Technology Readiness Level), and economic (e.g., capital expenditure, operating costs, and financing). For example, electrolytic hydrogen solutions need to be considered in the context of available clean electricity given anticipated buildout rates and land-use restrictions.
- Incentives should favour the lowest cost decarbonisation pathways for each facility (i.e., to avoid perverse incentives to use e.g. hydrogen when cheaper alternatives may exist).
- Coordination should be encouraged between co-located industries to deliver shared solutions and infrastructure.
- Government must work with industry to develop a solution for dispersed facilities with limited access to infrastructure. By providing policy clarity and stability over the longer-term, for example on plans for the transport and storage of CO2, and on end use applications of hydrogen, Government can help to attract private investment.
- Government has announced positive plans to accelerate the build out of key infrastructure projects, including through the development of a transmission acceleration action plan, connections action plan and Strategic Spatial Energy Plan. Policymakers must continue to streamline the permitting of new technologies, reduce administrative burdens, reform regulation, and ensure the join-up of relevant Government departments and regulators to speed up deployment – the role of the Electricity System Operator will be critical here. A lack of adequate planning processes has the potential to present a policy “valley of death,” potentially inhibiting the effectiveness of incentives in achieving climate targets.
- Planning processes can transform simple pledges and targets into reality by being:
- Proactive and risk-aware: engage in structured system-risk-management approaches to mitigate risks and develop robust options-based strategies, ensuring multiple pathways to success.
- Multi-jurisdictional, cross-sectorial, and with systems-perspective: identify opportunities to scale solutions across regions, benefiting from economies of scale and lower costs.
- Co-created with all stakeholders: directly tackle planning challenges and potential conflicts, fostering a sense of shared ownership and accountability among all parties.
- Based on comprehensive, transparent, scenario-based analysis: ensure efficacy and relevance.
- Actionable roadmaps, accountable progress tracking, adaptive learning: provide opportunities for continuous review, refinement, and adaptation, grounded in legal frameworks to compel action.
Background
Decarbonising the industrial sector will be crucial for the UK to achieve its emissions reduction targets. However, the sector can be challenging to decarbonise due to the hard-to-abate nature of some activities, e.g., where emissions need to be reduced by changing feedstocks or processes (e.g., steel, cement) rather than switching to low-carbon energy sources.2
To reach net zero emissions by 2050, industry is expected to rely on a mix of technologies3, including:
- Electrification, by switching from fossil fuels to electrified processes, based on the assumption that the power system will be increasingly decarbonised towards 2050. The highest potential for electrification is in low-temperature heat processes, but due to the long lifetime of heat equipment, and cost-optimisation planning to avoid stranded assets, the electrification of industrial end uses could be slower than in other sectors4.
- Electrolytic hydrogen, which can be used both as a clean fuel (e.g., in furnaces) and as an industrial feedstock (e.g., for ammonia). It can also reduce emissions from industrial production processes.
- Biomethane, which is the purified form of raw biogas, and which can be used as a natural gas substitute for high-temperature heat generation, hydrogen production, or as feedstock in the chemical industry5.
- Carbon capture and storage, including CCS-enabled hydrogen, for hard-to-abate sectors where the nature of the production process chemistry means that emissions will be significant even if heat is generated without emissions. One prominent example is cement, where process emissions from the calcination of limestone make up about two-thirds of the global emissions of the cement industry and are among the hardest to abate6.
Strengthened focus on innovation policies, with comprehensive funding and support for clean technologies from demonstration to deployment
Reaching the goals of the Paris Agreement requires the international community to employ the full range of tools capable of delivering zero- or very-low-carbon energy. The UK has a strong commitment to exploring all potential solutions, with UK Government funding currently supporting a range of technologies.
Whilst the majority of UK Government funding supports deployment, the Government must ensure support for later-stage activities to support commercialisation. Policy should bridge the gap between demonstration, deployment, and commercialisation to create a business case to scale up climate beneficial technologies within the UK. Innovative technologies such as greenhouse gas removals and nuclear fusion must be funded beyond research and development and demonstration, to ensure they can progress to deployment.
The US framework enabled by the IRA has become attractive for companies as the instruments beyond support for R&D help de-risk investments by including investment incentives and tax credits, which are crucial for deployment. Production credits are self-activating policy instruments, particularly important for the expansion phase, which give clarity and security over which projects will qualify for what kind of incentives ahead of time. To enable technology development and create industries, it is important that policymakers make use of various instruments across the different technology readiness levels. In the expansion stage, credits that are self-activating are especially important. Instruments that provide support for CAPEX include investment tax credits, loans, and grants, whilst those that provide support for OPEX include production tax credits, contracts for difference, and feed-in-tariffs. Other funding options include venture capital and government procurement.
Captura y almacenamiento de carbono
Carbon capture and storage (CCS) is a technology that will be needed for the UK to reach climate neutrality. The UK Government has laid out an appropriately ambitious timeline for CCS scale-up, targeting 20-30 million tonnes of CO2 captured and stored by 2030, and 50 million tonnes by 2050. Two CCS clusters are targeted by the ‘mid-2020s’ and a further two by 2030. To support this deployment, business models based on contracts for difference are being implemented for each sector that requires CCS, as well as a regulated asset base approach to transport and storage infrastructure. However, owing to delays in implementation of these policies, the UK is not currently on track to meet these CCS targets. Contracts are still being agreed with the first CO2 capture projects (Track 1, Phase 1), with Final Investment Decisions (FIDs) to be taken later this year. These projects will likely start operating by late 2027 at the earliest.
The £20 billion funding envelope agreed by Government is a positive development, but this is only expected to cover the eight shortlisted Track 1 Phase 1 projects. There is therefore considerable uncertainty as to the funding model and business case that will be available to future capture projects, including Phase 2 projects in Track 1 clusters, all projects in Track 2 clusters (Viking and Acorn), and potential projects in other industrial clusters and dispersed sites across the UK. There are several industrial areas in the country that will require CCS to decarbonise but do not have a clear route to access CO2 storage, notably the South Wales cluster and the cement and lime plants of the Peak District. While non-pipeline transport of CO2 is likely to play a role for these areas, the status of these forms of transport in CO2 infrastructure regulation (and their eligibility for funding) is yet to be clarified. The North Sea Transition Authority award of 28 offshore storage licences to date is also a positive step, but there is currently no real prospect of most of these licences being exploited in the near term, with no business case for CCS beyond the Track 1 clusters.
Given the limited political appetite for subsidising CCS deployment beyond this first wave of projects, there is a clear need to ensure an enduring incentive framework is in place for decarbonised industry, power, and greenhouse gas removals (GGRs). The carbon price signal provided by the Emissions Trading System (ETS) will underpin such a framework, but will need to be supported by the development of markets for low-carbon products and services, in which pricing reflects the cost of decarbonisation.
The UK has an opportunity to accelerate a critical technology which can reduce large amounts of CO2 emissions at low cost. Building a CO2 transport and storage infrastructure network is a no-regrets option to significantly reduce the emissions the UK produces currently and in the future, while also paving the way for negative emissions to reverse historical CO2 emissions. Policymakers have an opportunity to transform British industries, put them on the pathway to climate neutrality, and deploy a technology which will also be crucial at the international scale.
Key Policy Recommendations
- Accelerate negotiations for Track 1 projects to ensure that Final Investment Decision is reached by September.
- Clarify the funding model and business case for future capture projects, including for dispersed sites.
- Implement a plan, with clear milestones, to rapidly accelerate the build out of CO2 transport and storage infrastructure and promote interoperability of networks.
- Provide further clarity on the role of non-pipeline transport (rail, road, shipping) of CO2 in UK cluster expansion.
- Amend CO2 infrastructure regulation to include the status of non-pipeline transport of CO2 and clarify project eligibility for funding.
- Ensure an enduring incentive framework is in place for decarbonised industry, power, and GGRs, including the development of markets for low-carbon products and services.
- Maximise the economic opportunities presented by the storage of CO2 from Europe by minimising divergence between EU and UK CO2 storage legislation and regulation.
- Remove barriers to the import of CO2 for storage to the UK from the EU, due primarily to the separation of the UK ETS.
- Establish a long-term incentive for the deployment of permanent, CCS-enabled GGR, such as integration into the UK ETS with appropriate safeguards.
- Implement a ‘producer responsibility’ policy, such as a carbon takeback obligation (CTBO), to place a greater imperative to act on oil and gas producers and help ensure that storage is developed ahead of demand.
- Identify and share best practices with key international partners for national funding schemes, regulation and policies supporting carbon capture and storage.
Background
¿Qué es la captura y almacenamiento de carbono?
CCS is a solution which can eliminate CO2 emissions from processes where CO2 is generated by fossil fuels, biomass or feedstocks. It involves the separation of the CO2 from other gases, with the CO2 being captured, compressed, and transported to geological storage sites. It is then stored deep underground in porous rock formations, covered by an impermeable cap rock that effectively traps the CO2 in place. The overwhelming consensus among scientists is that geological storage of CO2 is permanent, with the injected CO2 staying trapped in the subsurface for millennia.
The risks of CO2 leakage are extremely low, with recent analysis from the UK Government showing that even in a worst-case scenario over 99.92% of CO2 will be contained in storage sites permanently. Moreover, even in the case of leakage, effects on the environment and climate are minimal, particularly when storing CO2 offshore.
Carbon capture technologies have been used safely and effectively for almost 100 years, while CO2 storage has been done for over 50 years. There are more than 20 commercial-scale carbon capture facilities operating globally, permanently capturing and storing around 40 Mt of CO₂ annually. Carbon capture and storage works at scale but has not been deployed sufficiently due to a lack of adequate policy or regulatory measures such as an effective carbon price. However, as greater steps are being taken to reduce global CO2 emissions, there are now over 500 projects in planning globally, including dozens across Europe.
Why does the UK need carbon capture and storage?
In its Sixth Carbon Budget report, the Climate Change Committee’s ‘Balanced Pathway’ relies on nearly 100 million tonnes of CO2 to be captured and stored by 2050 for the UK to achieve its legally binding goal of net zero emissions. Over half of this contribution is associated with the permanent removal of CO2 from the atmosphere, which will be essential both to balance any residual ‘hard-to-abate’ greenhouse gas emissions at net zero and to reverse the legacy of historic emissions. 22 million tonnes of CCS and CCS-enabled greenhouse gas removals are required by 2030 in this pathway, highlighting the challenge to scale up this technology in this decade: today, there is no CCS operational in the UK. In short, there is no climate neutrality in the UK without CCS.
Figure 4: CCS deployment in the Climate Change Committee’s favoured ‘balanced pathway’.7
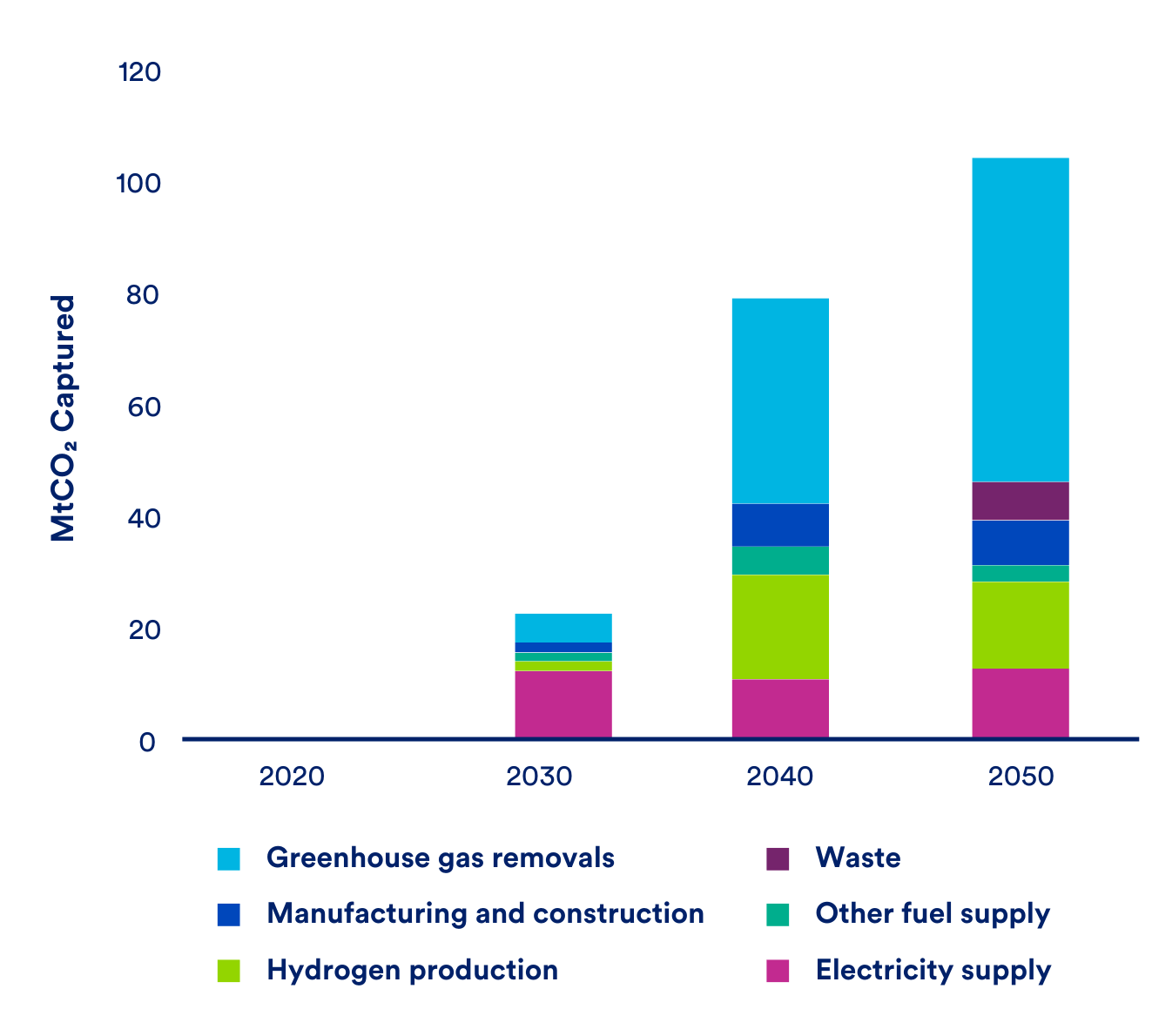
The carbon capture opportunity: A low-cost solution for a climate neutral industry
Carbon capture and storage is vital to decarbonise hard-to-abate industrial sectors, such as chemicals, fertilisers, steel or cement, which require high-temperature heat or inherently produce carbon dioxide as part of the production process.
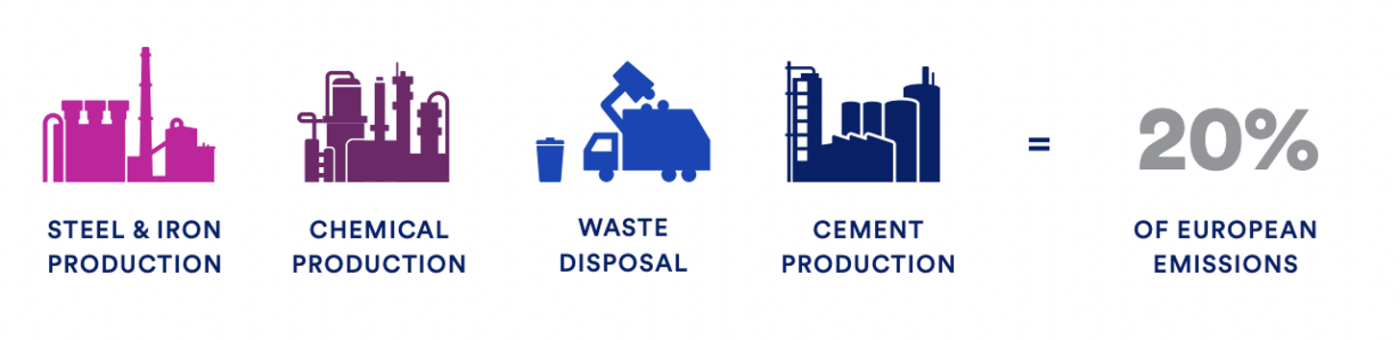
For cement, lime, some chemicals, and waste incineration, CO2 is emitted as an inevitable part of the process rather than from fossil fuel combustion. For example, about 60% of the emissions from cement are integral to the process chemistry and cannot be reduced by switching to low-carbon forms of energy. They result from heating the limestone, and that process releases CO₂. So, even if you could provide heat with renewable electricity, 60% of the emissions will remain. This is also true for waste incineration and chemicals production. For other industrial production processes like steel, various pathways to decarbonise exist, many of which involve the use of CCS either directly or indirectly – such as through the production of blue hydrogen. Nevertheless, the most challenging issue for many alternative pathways will be the availability of abundant, clean electricity and heat, particularly in the near term. CCS-based solutions can offer high volume cuts in industrial emissions by 2030.
Scaling carbon capture, removal, and storage is also crucial for the transformation towards a hydrogen economy, while it may also provide a source of clean, dispatchable power to complement the role of renewables in electricity systems. BEIS modelling to support the Net Zero Strategy identified a need for around 10 GW of CCS-equipped gas power plants by 2035. The geological storage of captured atmospheric or biogenic CO2 provides an indispensable means of removing CO2 from the atmosphere in a way which is durable, measurable, and permanent on climate-relevant timescales.
Rapid developments in alternative decarbonisation technologies or materials may diminish the need for CCS in future. However, we cannot afford to take the climate risk of assuming this will be the case. Current climate modelling already relies on ambitious assumptions for renewable energy growth, demand reduction, and innovation. Targeting the buildout of a CCS industry at significant scale (~10s of Mt) for 2035 is a ‘no regrets’ approach, which will ensure the UK’s decarbonisation trajectory is resilient to a range of future outcomes.
A responsibility to scale CCS globally
The latest IPCC Working Group III’s Contribution to the Sixth Assessment Report (AR6)8 includes carbon capture and storage as a critical technology in nearly all mitigation pathways. While pathways which do not rely on carbon capture and storage exist, they rely on unrealistic assumptions such as halving global energy demand in the next 30 years. The IPCC also stressed that currently, global rates of CCS deployment are far below those in modelled pathways limiting global warming to 1.5°C or 2°C, and that this delay is partially due to the lack of supportive policies. CCS will be necessary globally, but its deployment will first be required in climate-forward regions like Europe, so that the technology will be available at lower costs for developing economies in the future.
Ensuring the survival of British industries
As free allowances are phased out from the ETS, it is critical to ensure that these industries have the tools necessary to decarbonise rapidly. Policymakers must step in and ensure the widespread availability of the required innovative technologies to decarbonise, which will include carbon capture and storage for many industries. If decarbonisation is not possible, industries will either have to pay the carbon price or move their industries elsewhere.
The cement and concrete industries directly employ 74,000 people and indirectly support an additional 3.5 million jobs, primarily in the construction sector, while contributing around £18bn to the country’s GDP.9 The steel industry supported 33,400 jobs in 2019.10
Carbon Capture and Storage: A cost-effective climate solution for industry
Among the various technology pathways to produce clean industrial products like steel, cement, and chemicals, pathways which use CCS are among the cheapest. Figure 5 illustrates pathways outlined by the International Energy Agency (IEA) to produce low carbon industrial products under various pathways, showing those which use CCS are among the cheapest.
Figure 5: IEA estimates of levelised costs of producing low-carbon cement, steel, and ammonia via different low-carbon routes. Note: NZE denotes a hydrogen cost in line with the IEA’s ‘Net Zero Emissions by 2050’ decarbonisation scenario; 2022 represents the impact of higher gas prices.11
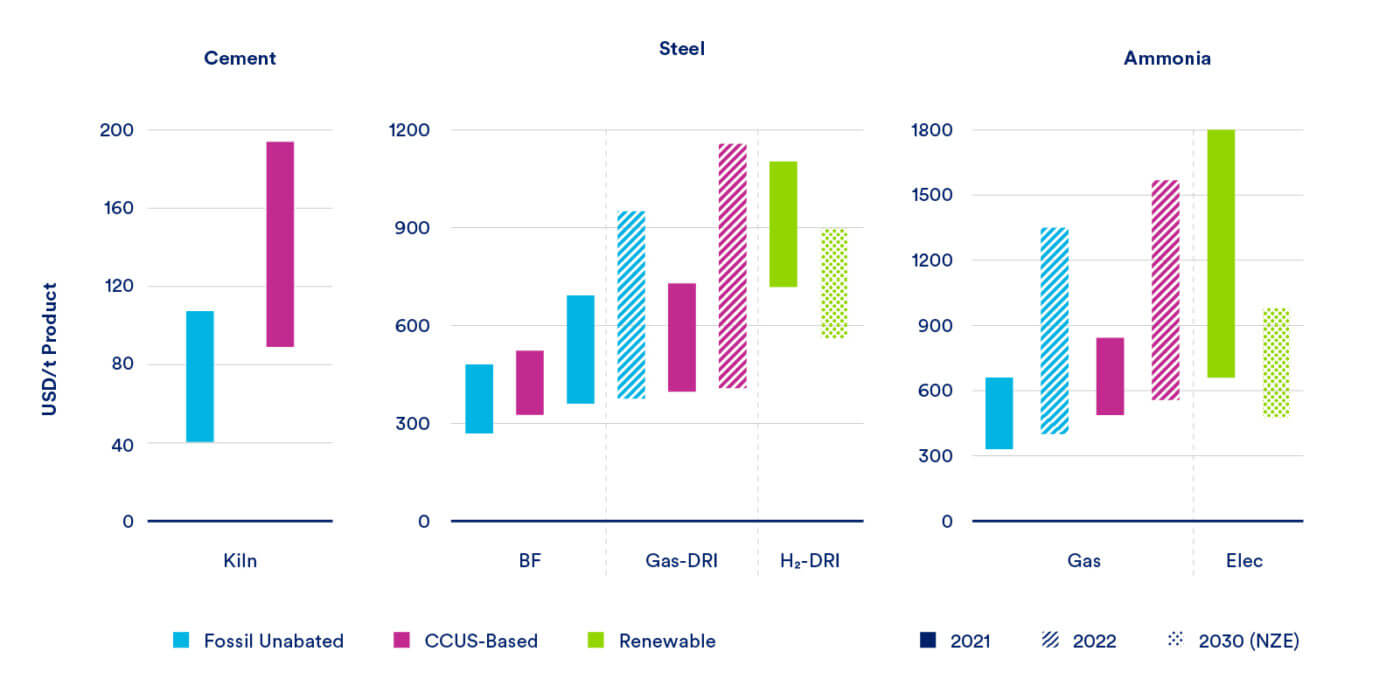
It is also important to note that while the additional cost increase of using CCS and other technologies to produce low carbon products may seem large, the additional cost to end-consumers is much less significant and therefore easier for consumers to absorb when applied to end-use products such as cars or buildings. For example, Figure 6 illustrates the additional cost of construction of a bridge using decarbonised cement and steel produced with CCS, resulting in an overall increase of just 1% to the total construction cost.
Figure 6: The added cost of a bridge construction using steel and cement produced with CCS which results in an additional cost of 1% and an emissions reduction of 51%.
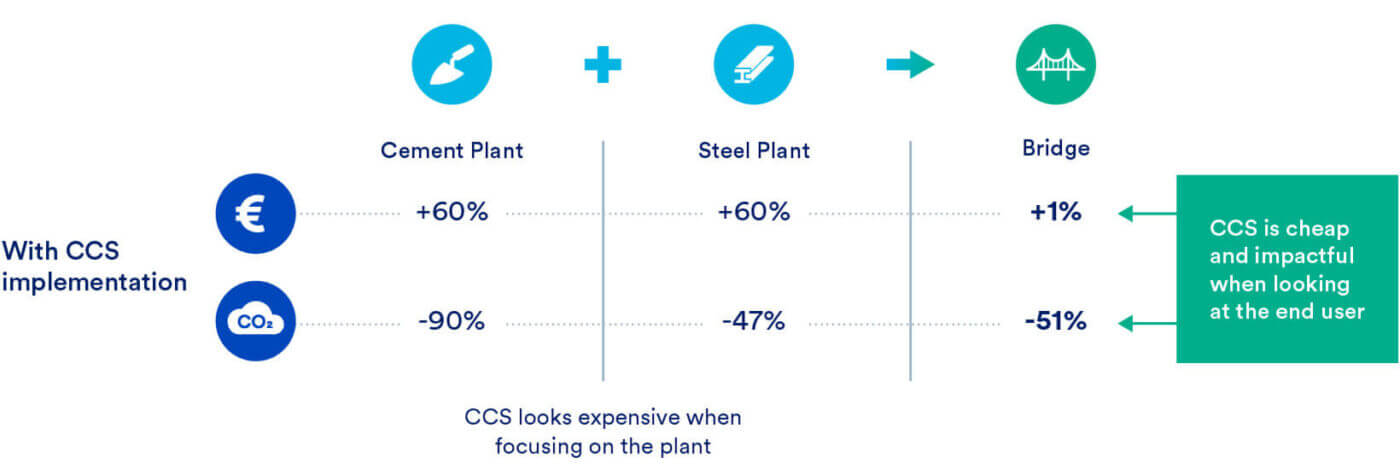
The need for CO2 infrastructure
The cost of CCS hinges upon many factors, especially the availability of CO2 infrastructure and sufficient storage capacity. In Europe, the key barrier to CCS development has been the development of storage sites. CATF has developed a tool, shown in Figure 7, which estimates the total cost of carbon capture, transport and storage for all emitting sources in Europe. The cost estimates are based on a range of scenarios including current storage site developments in Europe, the majority of which are in the North Sea, an expansion of storage development to areas which are suitable for CO2 storage and the availability of new CO2 pipelines. Ultimately, European industries will only be able to equitably avail of CCS at lower cost with the widespread availability of CO2 infrastructure.
Figure 7: The estimated cost of CO2 transport and storage in Europe under different scenarios for storage and pipeline infrastructure availability
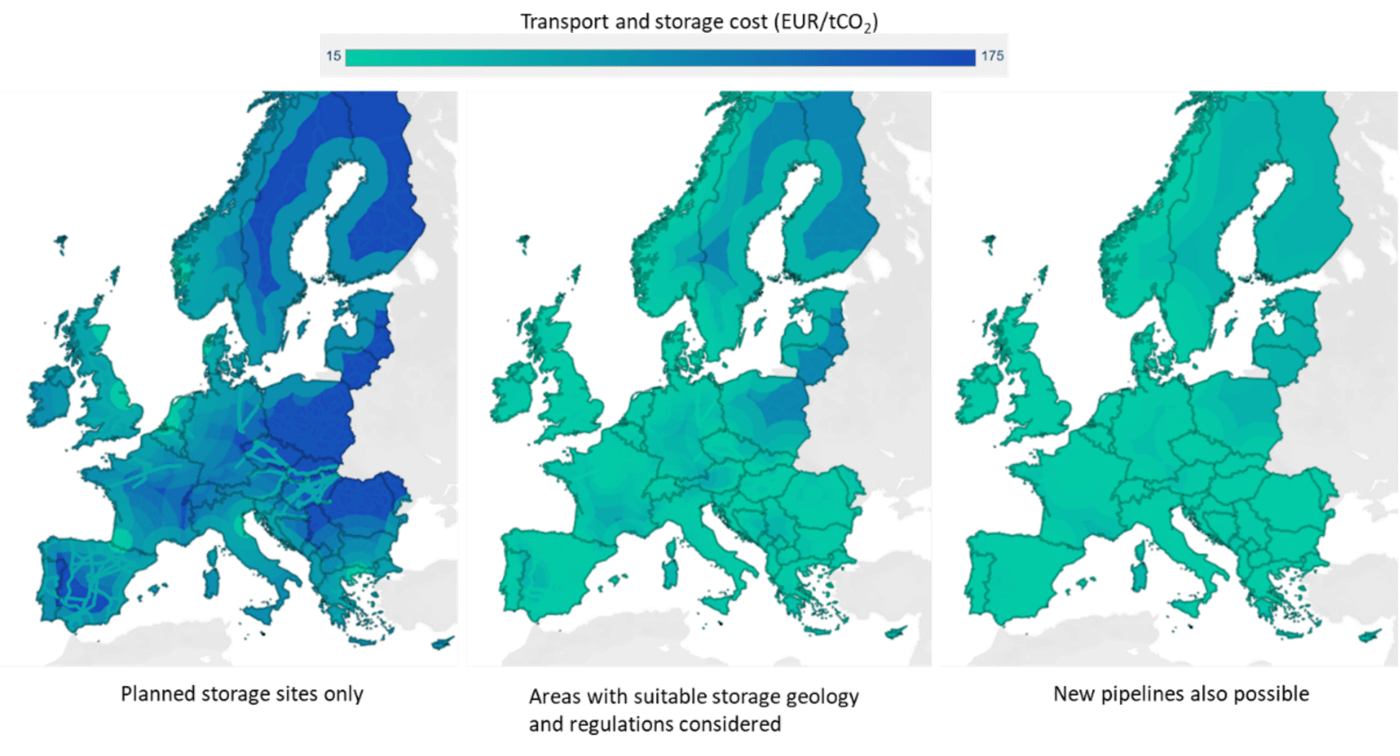
The UK has an unparalleled potential for geological storage of CO2 in Europe, with 78 Gt of theoretical capacity identified offshore (largely in the North Sea). In addition to decarbonising its own economy, the UK can therefore offer lower cost access to large-scale CO2 storage for EU emissions. Imported CO2 features significantly in the plans of several of the UK’s current projects and other recently licensed storage areas. While Norway also offers high-capacity storage sites and is rapidly developing import options for EU CO2, storage operators in the UK would have a competitive advantage due to their closer proximity. Pipelines proposed to link Belgium and Germany with Norway would be nearly 1000 km in length, while a potential Belgium-UK pipeline following the existing gas pipeline route would be less than a quarter of this distance. Both countries’ storage areas will ultimately need to be readily available for imported emissions if the EU is to reach its climate goals.
CATF modelling with Element Energy identifies a potential need for 100-240 million tonnes per year of CO2 storage in the North Sea Basin by 2050, depending on the buildout of storage elsewhere in Europe.12 Achieving the necessary storage build-out rates in the North Sea Basin will require the offshore resources and expertise of all North Sea countries to be employed in parallel and in a coordinated manner.
However, regulatory barriers currently prevent the import of CO2 for storage to the UK from the EU, due primarily to the separation of the UK ETS. There is a risk that this will be compounded by further divergence of CO2 storage legislation. To address this, the EU and the UK could agree a Memorandum of Understanding which mutually recognises both Parties’ CCS standards, so that for ETS purposes, CO2 storage in each jurisdiction is recognised by both ETS systems. The principle of equivalence (an EU concept meaning that the regulatory or supervisory regime of country B relating to a particular area is determined to be of an equivalent standard to that which applies in country A) could provide a way to achieve mutual recognition.
The role of producers
The oil and gas sector has the expertise, data, and assets to support the development of carbon capture and storage in the UK. With record-high profits from 2022, the industry also has the financial resources to ensure CO2 storage capacity is developed at scale and on schedule for the country’s decarbonisation goals. Under the current ‘emitter-focused’ business models for CCS being developed in the UK and around Europe, CO2 storage development has proved slow, given the long development times for these projects and the need for investors to have contract certainty from CO2 capture plants before taking Final Investment Decision.
A ‘producer responsibility’ policy, such as a carbon takeback obligation (CTBO), can place a greater imperative to act on oil and gas producers and help ensure that storage is developed ahead of demand. The EU’s Net Zero Industry Act (NZIA) includes such an obligation (to provide capacity) on oil and gas producers, while a form of CTBO in the UK has been called for by the Net Zero Review and the All-Party Parliamentary Group on Net Zero. Unlike the capacity obligation in the NZIA, a CTBO would require oil and gas producers to source and store a proportion of the carbon they produce. Such a policy could help kickstart storage development in the UK while also providing an enduring incentive framework for CCS, ultimately ensuring that a 1.5°C-compatible net zero is achieved. Government should give serious consideration to how a form of CTBO could be implemented in practice, particularly in light of recent announcements on the expansion of North Sea oil and gas production. Government must ensure that expanded production is linked to equally ambitious goals for the decarbonisation of fossil fuel use.
Greenhouse gas removals
As the IPCC highlights, achieving the 1.5°C climate target will require negative emissions through Greenhouse Gas Removals (GGRs). Whilst deep emissions cuts must remain the core strategy due to their cost-effectiveness and immediate impact, even with deep and sustained cuts residual emissions will persist that must be balanced through carbon removals. The UK’s Committee on Climate Change estimates that 43.5-130 MtCO2/year of GGRs will be needed by 2050 to address unavoidable emissions.
GGRs can play a number of roles in supporting and achieving the UK’s climate goals. They can:
- Mitigate near-term emissions: engineered GGRs like bioenergy with carbon capture and storage (BECCS) and direct air capture with carbon storage (DACCS) offer immediate impact on atmospheric CO2, complementing emissions reductions and removals from nature-based solutions such as afforestation and reforestation.
- Counterbalance residual emissions: these technologies can directly address emissions from hard-to-abate sectors, crucial for achieving and sustaining net zero.
- Reverse legacy emissions: GGRs can permanently remove legacy fossil fuel emissions, contributing to long-term climate stabilisation.
Key Policy Recommendations
- Accelerate existing proposals by streamlining permitting processes and providing targeted financial support to expedite the launch of proposed and new engineered GGR projects.
- Explore the integration of GGRs into the UK’s ETS, while being conscious of the risks involved.
- Define the process for issuing GGR credits under government business models.
- Develop standards and monitoring, reporting and verification (MRV) methodologies for all engineered GGR technologies in the scope of government support.
- Collaborate with stakeholders to establish clear and consistent standards for the specifics of engineered GGRs in terms of the transport and storage of CO2.
- Define clear liability structures for any potential reversals of CO2 from leakage of storage associated with engineered GGR projects.
Background
While the need for greenhouse gas removals is clear, it is fundamentally easier, cheaper, and more effective to prevent an emission than to subsequently remove it, given the immediate climate impact of atmospheric CO2 and the energy requirements of greenhouse gas removals. Emphasising emissions reduction strategies such as energy efficiency and renewable energy deployment remains the most cost-effective and immediate way to reduce emissions. This “reductions first” approach minimises future reliance on engineered GGRs and ensures a more sustainable and efficient long-term solution.
Currently, the two most technologically advanced engineered GGR methods are BECCS and DACCS. The geological storage of CO2 derived from either of these processes offers a means of permanently removing large volumes of carbon from the atmosphere. Any engineered greenhouse gas removals must be complementary to emissions reductions and removals by the land sector. Overreliance on any one removal method poses significant risks, due to the uncertainties surrounding the deliverability, availability, scalability, mitigation potential, MRV challenges and possible negative externalities.
Unlocking the potential of engineered GGRs in the UK
While the UK government has recognised the importance of engineered GGRs, concrete action has lagged behind ambition. As of now, the UK has no operational large-scale engineered GGR projects, despite promising proposals like the Drax BECCS plant and the Sizewell C DAC project. This gap impedes progress and learning opportunities which can unlock potential cost reductions. While policy proposals such as the GGR business models are commendable, translating them into concrete action and implementation is crucial to meet 2030 milestones and avoid falling behind global leaders. The UK Government should accelerate existing proposals by streamlining permitting processes, providing targeted financial support to projects, and offering clear policy signals to expedite the launch of proposed BECCS and DACCS projects.
While the UK government has begun the process of exploring the integration of GGRs into the UK’s emissions trading scheme (ETS), integrating GGRs is unlikely to be sufficient to scale these technologies on its own. The current UK ETS price does not reflect the true cost of greenhouse gas removal, particularly for DACCS, which has an average removal cost of £786 per tonne compared to the UK ETS price of around £34 per tonne. This price differential is indicative of the long way that these technologies must go before being cost competitive, and highlights the need for further research and innovation support, as well as policy mechanisms such as targeted grants, feed-in tariffs, or contracts for difference specifically for engineered GGR projects to bridge the cost gap and attract private investment.
Nonetheless, carbon credits generated by engineered GGRs could possibly be gradually incorporated into the ETS to provide a driver for the sector, moving away from the poorly regulated voluntary markets that drive deployment today. Government support is vital for ensuring sufficient demand for technologies as they mature in commercial viability. It will be crucial that the government address the price limitations and political volatility of the ETS to provide an appropriate long-term market for engineered GGRs.
In tandem with targeted policy support a regulatory framework that can serve as the foundation for this emerging industry will be crucial. The government should define the process by which GGR credits can be issued under government business models. This includes developing standards and MRV methodologies for all engineered technologies in the scope of government support. It is recommended the government develop new methodologies specific to technologies to raise credibility on GGR accounting, however, a combination of new and existing methodologies could be developed for adoption should they be consistent with current GGR standards, including those of a third party. Monitoring, reporting and verification (MRV) methodologies will be crucial to the development of engineered GGRs in the UK. Collaboration with international partners and building from the base of existing knowledge can help to develop robust and internationally recognised methodologies for measuring and accounting for CO2 removal achieved through different engineered GGR technologies.
The UK government should develop engineered GGR-specific standards through collaboration with stakeholders to establish clear and consistent standards for the specifics of engineered GGRs such as captured CO2 purity, storage permanence, and environmental impacts, ensuring standardisation and the environmental integrity of engineered GGR projects. Defining clear liability structures for any potential reversals of CO2 from leakage of storage associated with engineered GGR projects can provide clarity for project developers and assurances to the public and government that CO2 is being permanently stored.
Low carbon hydrogen
Low carbon hydrogen will be required to decarbonise sectors of the economy where no other energy-efficient or cost-effective decarbonisation options are available. These include energy-intensive industries, such as oil refining, petrochemical, steel, and ammonia production, as well as segments of the heavy-transport sector. A comprehensive framework and market tools must be in place to guarantee reliable and constant hydrogen supply to these industries.
Figure 8: CATF priority ranking of potential clean hydrogen end use sectors
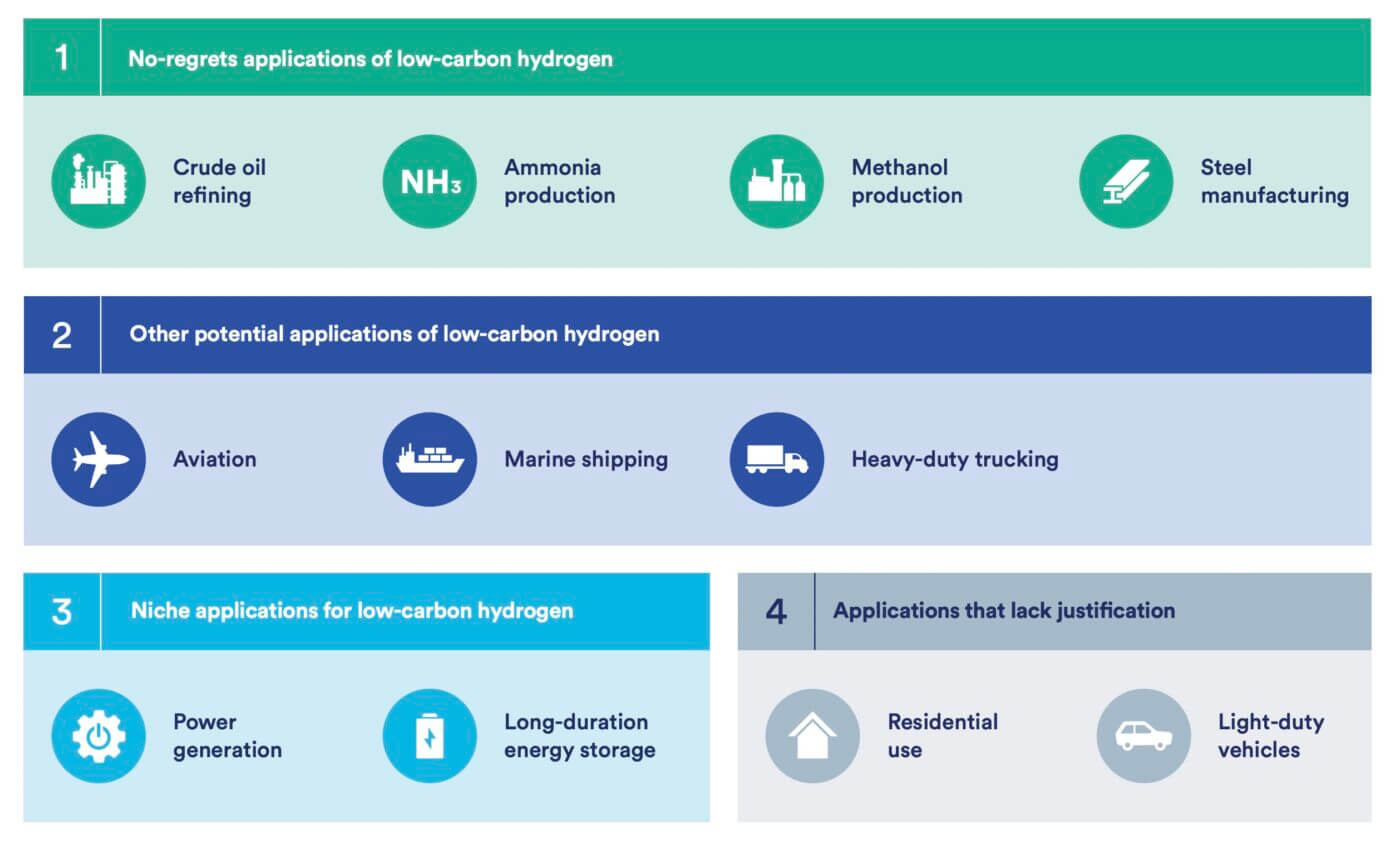
Figure 8 outlines the key end use sectors for which low carbon hydrogen will be crucial. Government should outline these priority sectors and ensure that the limited volumes of low carbon hydrogen that will be available over the coming years are directed to their decarbonisation first.
Key Policy Recommendations
- Set a strategic decision on where low carbon hydrogen will be deployed, prioritising ‘no-regrets’ sectors first, where limited or no other energy-efficient or cost-effective decarbonisation options are available. Such sectors include refining, (petro-)chemicals, ammonia and methanol production, and primary steel manufacturing.
- Focus on segments of the transportation sector where low carbon hydrogen is needed the most, including as feedstock and fuel for aviation, marine shipping, and heavy-duty road transportation.
- Rule out the use of low carbon hydrogen for blending into the gas grid and for home heating.
- Outline a clear roadmap to facilitate investment in the production of hydrogen and site low carbon hydrogen production as close as possible to priority off-takers.
- Assess the future for low carbon hydrogen demand realistically and determine how much of this can be met from domestic production vs. what will need to be met by international imports of low carbon hydrogen and its derivatives (e.g., ammonia).
- Plan carefully for the infrastructure needed to facilitate any needs-driven hydrogen imports, transportation and storage to ensure that it is both cost-effective and energy efficient.
- Align the UK’s low carbon hydrogen certification scheme with other international standards to facilitate imports and exports of hydrogen across borders, including the full scope of associated upstream emissions in the greenhouse gas accounting methodology.
Background
As part of the future decarbonised energy mix, low carbon hydrogen has emerged as a technology to enable global decarbonisation, particularly with its application in some of the most hard-to-abate sectors. The term ‘low carbon hydrogen’ covers the full spectrum of clean hydrogen pathways that yield limited greenhouse gas emissions.
Hydrogen is already produced and consumed in high volumes today – around 95 million tonnes globally in 2022 – and is used as a crucial feedstock and fuel in heavy industry processes that supply many of society’s essential commodities. However, it is rarely found in a naturally abundant state and therefore must be liberated from a compound form. Most of the hydrogen produced globally today is via steam or autothermal reforming (SMR/ATR) of methane (CH4) from natural gas, with a smaller fraction produced through coal gasification. Today’s hydrogen typically has very high associated emissions due to the process of producing the molecules, emitting almost 1 Gt of CO2 per year.
To make hydrogen low carbon, producers can install carbon capture technology and impose strict methane emissions controls onto the existing SMR/ATR facilities. Alternatively, they can use water-based (H2O) electrolysis powered by clean firm or renewable energy, such as wind, solar, nuclear, or other emerging technologies (such as superhot rock geothermal), but less than 1% of all hydrogen produced today is made from such low carbon pathways.
Low carbon hydrogen provides an essential tool for reducing emissions in certain sectors but is far from a silver bullet for decarbonisation and should not be deployed indiscriminately to all sectors as if every potential end-use has equal merit. Policymakers should instead recognise that hydrogen is a critical chemical for running some of today’s industries sustainably, feeding future populations (as a feedstock for ammonia and fertiliser production), and fuelling certain modes of transportation that underpin the global economy.
The limits of relying exclusively on renewable hydrogen
The UK’s target of up to 10 GW of low carbon hydrogen production capacity by 2030, with at least half of this from electrolytic hydrogen (powered by either renewables or new nuclear), is thought to be at the lower end of what is needed. However, using scarce, renewable electricity to produce hydrogen while the grid is not fully decarbonised is a counterintuitive approach to resources deployment, particularly when the electricity consumption is expected to increase as electrification gains momentum to reduce emissions. Renewable electricity deployment should be prioritised to decarbonise the electricity grid, with the opportunities for renewables-powered ‘green’ hydrogen increasing when renewable electricity production is abundant.
Moreover, scaling renewable-powered hydrogen faces two major issues: (1) the limitations of deploying renewables at scale and (2) the limitations of manufacturing electrolysers at scale. If renewable-powered hydrogen is not available in substantial quantities to fully support forecasted UK hydrogen demand, efficient deployment of hydrogen applications based on proven technologies (such as SMR or ATR reforming with CCS and strict upstream methane controls) should be considered a key intermediary solution to rapidly ramp-up low carbon hydrogen production capacity at scale and bridge any existing gaps.
Hydrogen imports
Owing to its physical properties, hydrogen is a difficult molecule to transport, therefore low carbon hydrogen production should be located as close to end users as possible. Any hydrogen transport should (a) be limited to cases where hydrogen serves a very specific need and (b) use the most energy- and cost-efficient methods, such as pipelines.
CATF investigated the techno-economic pathways of importing hydrogen into Europe from global locations, including Norway, the US, the Middle East and North Africa (MENA). Under all scenarios, importing large quantities of hydrogen over long distances will be an expensive and relatively energy inefficient endeavour due to the inherent properties of hydrogen, particularly its low volumetric density. Of the options available, the results found that the most cost-effective method to transport hydrogen is by pipeline, ideally over the shortest distances possible, followed by maritime transport in the form of ammonia for direct use. However, if the ammonia is ‘cracked’ to liberate pure hydrogen, this incurs significant energy penalties making the process even less efficient and costly.
Imported ammonia should be prioritised for industry requirements for ammonia specifically, such as for fertilisers and chemicals production, and as a zero-carbon fuel to decarbonise maritime shipping, as the fuel is much cheaper and more stable to transport via ship and truck than hydrogen. To ensure ammonia is low carbon, it must be made using a low carbon hydrogen feedstock. If the UK decides to pursue the use of low carbon ammonia as a shipping fuel, it must ensure that it does not draw available volumes away from other present day ammonia applications (e.g., fertiliser production), particularly in the near term.
Where connecting hydrogen infrastructure across borders is needs-driven, the UK should approach international collaborative efforts carefully, considering the logistics and cost-effectiveness of such large-scale hydrogen transportation, considering where and how hydrogen and ammonia will be imported and exported and where it is needed for deployment. If hydrogen must be transported over longer distances, new infrastructure may be needed, which will come with long time horizons and significant costs for planning and build out. The UK should seek to utilise any existing assets and the most efficient transportation methods to help streamline the process and avoid cost ventures or stranded assets.
Furthermore, a net zero UK in a world that has not achieved significant emissions reductions will not be sufficient to address the global challenge. Any low carbon hydrogen imported to the UK should not take place at the expense of decarbonisation in other parts of the world, particularly in regions faced with energy poverty, who require domestic resources for their own domestic purposes, such as renewables build out for clean energy electrification or low carbon hydrogen production for supplying clean fertilisers to their own agriculture industry.
Unavoidable low carbon hydrogen
Certain energy-intensive industries, such as refineries, which produce feedstock for the chemicals industry, will require low carbon hydrogen produced with installed carbon capture facilities to decarbonise because of their technical configuration. Some of the hydrogen used in these industries cannot be replaced with exogenous hydrogen because it is supplied as a by-product from internal industrial processes, and altering this supply would risk disruption to complex value chains. These industries also require a constant, reliable hydrogen supply and cannot accommodate intermittencies, which could result in significant financial losses and interruptions to operations.
Additionally, refineries produce off-gases that are currently used to generate heat. These molecules can only be decarbonised through the production of low carbon hydrogen. Off-gases are mostly methane and ethane molecules that are directed to the facilities’ fuel gas system to generate high-process temperature heat. These molecules are mixed with natural gas to complete the refineries’ heat requirement and combusted in large ovens. To decarbonise these processes, industry can deploy carbon capture and/or convert the fuel gas into low carbon hydrogen. Therefore, the decarbonisation of these industrial facilities’ fuel gas by means of converting it into low carbon hydrogen is crucial for the refining and chemicals industry. Although in early demonstration phases, this is currently being done in the UK in the Stanlow Refinery.
CATF has estimated that the volume of low carbon hydrogen required to decarbonise the fuel gas of a high-complexity refinery of approximately 20,000 tonnes per annum of crude is in the range of 200,000 to 260,000 tonnes per year.
Decarbonisation timeline
Low carbon hydrogen can be scaled faster and with high-capacity factor and utilisation rates when compared to renewable hydrogen because of its high Technical Readiness Level. H-vision estimates that before 2025, low-carbon hydrogen production facilities can be deployed and fully operable, assuming that the CO2 infrastructure is available.
CATF has developed an example of low carbon hydrogen deployment to decarbonise refining assets in Europe’s largest port. Projects to convert off-gases into low carbon hydrogen will support the energy transition required to address the current emissions of these large industrial facilities. For this to materialise, there is a need for the development and deployment of the carbon dioxide transportation and storage infrastructure, as well as strong policy mechanisms such as contracts for difference to create an environment suitable for investment in these technologies.
Hydrogen end-uses and recommended prioritisation for deployment
Emissions must be reduced across the entire life cycle and value chain. Therefore, emissions totals need to be calculated and priorities based on this.
Entre los sectores prioritarios de primer orden para el despliegue del hidrógeno figuran:
- Crude oil refining
Almost 50% of all hydrogen produced today is consumed in petroleum refineries. Refineries produce a wide array of products critical to the functioning of today’s economy and hydrogen is a critical feedstock in their production. Many of these products are hard to replace quickly and economically, and they are likely to remain in our future economy as well. Hydrogen is used, for example, to remove sulphur, nitrogen, oxygen, olefins, and heavy metals in transportation fuels. Hydrogen also plays a role in increasing the product yields from hydrocracking operations and to produce a variety of non-fuel products such as lubricants and anode grade coke, a key component in the production of steel and aluminium. Using low carbon hydrogen to replace unabated hydrogen production in refineries could reduce the industry’s emissions by 240-380 Mt/year, equivalent to the total emissions of the UK.
- Ammonia production
Ammonia is a critical ingredient in nitrogen fertilisers which play an essential role in providing a secure food supply for human populations worldwide. In fact, 70% of global ammonia supply goes to fertiliser production13. Ammonia also has other important uses such as for explosives in the mining sector, synthetic fibres, and specialty applications. Hydrogen is an intermediate input in ammonia production, which involves reacting hydrogen with nitrogen from the atmosphere. Current ammonia production is estimated to generate almost 500 Mt per year of global CO2 emissions. Given the critical role ammonia plays in underpinning our modern agricultural system, decarbonising the carbon intensive hydrogen feedstock used in its production should rank high on the priority list for low carbon deployment.
- Methanol production
Methanol is a critical industrial chemical used to produce certain chemicals (e.g., formaldehyde, acetic acid) and plastics (methanol to olefins). Methanol and its derivatives are also used as fuel additives to improve combustion properties. Hydrogen is an intermediate input and is reacted with a carbon to produce methanol. Current production is estimated to emit over 100 Mt per year of global CO2 emissions. Given the importance of methanol in industrial sectors, decarbonising the hydrogen used to make methanol should rank high on the list of applications for low carbon hydrogen.
- Steel manufacturing
Hydrogen currently plays a role in steel manufacturing via the direct reduced iron-electric arc furnace (DRI-EAF) process, where hydrogen from a synthetic gas (mainly H2+CO) is used to remove oxygen from DR-grade iron ore. The idea of using low carbon hydrogen in existing DRI applications has been proposed as a pathway for reducing emissions from steel manufacturing.
Los sectores clasificados como de segundo orden de prioridad para el despliegue del hidrógeno, debido a su estado incipiente, incluyen:
- Aviation
Decarbonising the aviation sector will require low carbon hydrogen to produce renewable diesel and kerosene – known more commonly as sustainable aviation fuels (SAF) – by hydrotreating biomass feedstocks, oils, and fats of biogenic origin. This can be done by upgrading biomass-based sustainable aviation fuels (bio-SAF), synthesising jet fuel from hydrogen and captured carbon (synthetic SAF), and, potentially, powering aircraft that directly utilise hydrogen fuel. SAF draw interest because they have the advantage of compatibility with existing infrastructure and engines (for this reason, they are often called ‘drop-in’ fuels). Using low carbon hydrogen in the production of biomass-based transportation fuels could help reduce associated lifecycle emissions. However, as highlighted in a CATF report, land-use and supply chain constraints on biomass feedstocks mean that other fuel options will need to be developed, including synthetic fuels (or ‘e-fuels’) produced using a combination of hydrogen, electricity, and CO2 sourced from non-biogenic feedstocks. Synthetic fuel production, however, is currently technically and economically challenging. If all flights between JFK and Heathrow airports were to run on e-fuels, for example, a facility the size of the NEOM Green Hydrogen Complex would be required just to supply the quantities of hydrogen needed to produce these fuels.
- Marine shipping
Low carbon ammonia is a strong contender as an alternative marine fuel. Health, safety, and environmental concerns associated with bunkering, storing and combusting ammonia would also need to be thoroughly examined before any large-scale use of ammonia can be cleared as a potential pathway for decarbonising marine transport on open seas. Another potential low carbon marine shipping fuel is methanol and many cargo ships being built today incorporate dual fuel capability to handle a future mix of marine oil and low carbon methanol. However, unlike ammonia, methanol emits CO2 at the point of combustion, so to produce a low carbon fuel, ‘sustainable’ carbon atoms would need to be sourced for the low carbon methanol production process.
- Long-haul transportation fuel
In road transport, long-haul hydrogen fuel cell vehicles may play an important role alongside battery electric vehicles in decarbonising the trucking sector. Implementation in Europe, however, will ultimately be determined by several factors, including cost, fuel and fuelling infrastructure availability and well-to-wheel lifecycle emissions.
Hydrogen is also being considered as a decarbonisation option for other sectors but in some of these cases it may not be the most suitable pathway. This is particularly true where other more energy- and/or cost-efficient options exist, such as electrification, CCS, and heat pump installation. Examples include:
- Power generation
There has been increasing interest in using low carbon hydrogen as a replacement to natural gas for power production since it emits no CO2 when burned. However, numerous technological, infrastructure, and system challenges would need to be addressed, for example the quantities of hydrogen needed would likely necessitate geologic storage and dedicated transmission and distribution pipelines. Associated costs make it important to focus on the carbon intensity of any hydrogen used. Two options present themselves – using hydrogen from natural gas with CCS and strict upstream methane emissions control or using hydrogen from electrolysis with clean electricity. For the former, combusting this low carbon hydrogen in a simple cycle power plant reduces lifecycle emissions from the power plant by roughly half compared to natural gas combustion. This combined with the cost of producing hydrogen using natural gas with CCS, however, results in CO2 abatement costs significantly higher than that of most decarbonisation options available in the power sector. Using electrolytic hydrogen, powered by low carbon electricity, is unlikely to be more appealing due to round-trip efficiency. 76% of the electricity used to make the electrolytic hydrogen is not recovered and in practical terms can be considered lost. Put another way, in a grid that is not already fully decarbonised, four units of clean electricity will be diverted from further grid decarbonisation to deliver one unit of clean electricity, effectively losing three units of clean electricity that could be used to serve other direct electricity end uses.
- Long-duration energy storage
The role that electrolytic hydrogen might usefully play in a decarbonised power system is as a form of long-duration energy storage for grid balancing at times when renewable generation would otherwise exceed demand and would need to be curtailed. However, this role is likely to be relevant only in a fully decarbonised grid. Even then, an evidence-based analysis would be needed to examine the entirety of the power system design, evaluate alternatives for long-duration energy storage, and optimise total system cost and decarbonisation pathways.
- Natural gas blending and residential use
Blending low carbon hydrogen into the gas grid, such as for deployment in home heating, would dilute the environmental benefits of a scarce commodity. Over 50 independent studies have concluded that decarbonisation alternatives for home heating, such as heat pumps, solar thermal systems, and district heating, are more economic and energy-efficient, and have a smaller environmental impact than hydrogen. Though routinely used in industrial applications, hydrogen’s use in residential settings presents potentially serious safety hazards, both due to hydrogen’s susceptibility to leakage and its ignition rage, which is six times that of natural gas.
- Light-duty vehicles
Hydrogen fuel cell vehicles (CEVs) require up to 2.5 times as much energy as electric vehicles and their cost per kilometre or mile travelled are multiple times higher. This is likely a key factor behind their limited sales and the small number of auto manufacturers with active efforts to develop a hydrogen passenger vehicle. The advantages that light-duty fuel cell electric vehicles currently offer relative to battery electric vehicles (longer range and shorter refuelling time) may be important to some users, but battery technology improvements will likely render these features less decisive in terms of favouring fuel cell vehicles.
Descarbonización del transporte
Transport emissions represent the largest source, 26%, of the UK’s total GHG emissions and continue to rise. Decarbonising transportation hinges on a massive increase in the production and uptake of electricity for light-duty cars and delivery vehicles and zero-carbon fuels (fuels that do not emit carbon when combusted) for aviation, marine shipping, and some heavy-duty trucking applications. Accelerating the introduction and deployment of zero emissions vehicles in all transport sectors will be co-dependent on the development of a robust network of charging and zero-carbon fuelling infrastructure, and economic incentives to underpin investment in low- and zero-emissions transportation systems and vehicles.
Key Policy Recommendations
E-fuels
- Provide tax or other production incentives and initially small then scaling market requirements for zero-carbon fuels. This will entice both producers and buyers to switch from conventional to alternative fuels and help bridge the notable cost gap.
- Boost Research, Development and Demonstration (RD&D) of innovative technologies that could aid in decarbonising transportation, including Direct Air Capture and advanced fuel cell types.
Shipping
- Adopt a target of zero-emissions by 2050 for the maritime sector, taking into account emissions across the entire lifecycle of different propulsion technologies.
- Develop zero-carbon fuel supply, bunkering and fuelling infrastructure at major ports, alongside the development of critical safety regulations.
- Increase ambition of e-fuels targets for shipping and incentivise the deployment of hydrogen-based fuels like ammonia while they are not yet competitive with conventional fuels.
Aviación
- While Sustainable Aviation Fuels (SAF) are often touted as a key strategy for reducing the aviation sector’s greenhouse gas emissions, the bulk of fuels under this umbrella are in fact biofuels that can have serious negative consequences for environment and climate, driving up the demand for commodity crops and motivating farmers to convert natural land into farmland. Demand for biofuels should therefore be further restricted to fuels made from waste matter and other environmentally sustainable feedstocks. Aviation should be prioritised for their uptake.
- Accelerate public and private investment in the innovation, development, and deployment of alternative, non-biogenic fuels and propulsion, which will be essential to eliminating emissions from aviation. ‘Blue’ hydrogen can help accelerate the production of Sustainable Aviation Fuel whilst meeting the desired emissions threshold – it can be scaled much faster, can be produced at lower cost today, and can have associated emissions as low as ‘green’ hydrogen.
- Address non-CO2 emissions from aviation, including by developing an effective Monitoring, Reporting and Verification system for the level of aromatics, naphthalene and sulphur in jet fuel.
Background
Decarbonising the UK’s transportation sector by mid-century will require a massive scale-up in the production and uptake of zero-emission electricity and zero-carbon fuels (fuels that do not emit carbon when combusted). While great progress has been made in battery-based electrification, especially for light duty vehicles; aviation, marine shipping, and some heavy-duty trucking uses will need alternative fuels to decarbonise. Between one quarter and one half of the transportation sector’s greenhouse gas emissions come from vehicles that will be difficult or impossible to power with batteries.
To scale up climate-aligned use of zero-carbon fuels, in addition to zero-emissions vehicle standards and low carbon fuel requirements, it will be critical for the UK to plan and scale the production and or importation, transportation, storage and fuelling infrastructure needed for each major transport sector. Developing this fuelling infrastructure will further entice producers and off-takers to switch from conventional to alternative fuels. More zero-emissions electricity, more transmission lines and more and higher-capacity charging are also needed to support the full spectrum of battery-electric vehicles.
While a variety of alternative fuels, and further innovations in fuel efficiency, will play a role in reducing emissions from different modes of transportation, there will be limits to how far some, such as bio-based fuels, can scale before having detrimental impacts, including driving higher emissions. It will be all the more critical for the UK, in cooperation with the US, the EU and other major trading partners, to assess the scalability of zero- or near-zero carbon fuels and prioritise the needed fuelling infrastructure, in particular for the marine and aviation sectors.
Nuclear
The UK Government has shown strong support for nuclear energy, with plans for a “revival of nuclear power” after many years of stagnation and delay. With the creation of Great British Nuclear, the UK has an opportunity to set out a clear pathway for nuclear energy going forward and rapidly expand the construction of new nuclear power plants in the UK, whilst growing necessary supply chains and developing a home-grown skilled workforce.
Key Policy Recommendations
- Existing planned projects, such as Hinkley Point C must be delivered, but the sector needs to be radically transformed to allow for reactors to be delivered on time and at much lower cost. This could be made possible by a mass-manufacturing model.
- British expertise in Small Modular Reactors (SMRs) should be harnessed to enable the UK to emerge as a leader in this field. Differentiating the regulatory and siting processes for new small modular reactors and advanced reactors will ensure these innovative technologies are deployed quickly and safely.
- Collaborative efforts to harmonise and standardise nuclear licensing and regulation internationally will be a cornerstone of expanding SMR deployment of UK-backed SMR technology overseas.
- Great British Nuclear can fulfil the function of a joint procurement platform to streamline development of new projects and increase transparency and buying power.
- Focus on developing a home-grown supply chain and skills will be particularly important as the UK is embarking on a path towards Great British Nuclear. Additional funding and support should be directed towards nuclear education, training regulators and operators, and providing apprenticeship opportunities in the nuclear sector.
Background
Scaling nuclear energy
Scaling nuclear energy to the levels required to meet climate goals will require a significant departure from business as usual, with a profound system change and disruption of the existing commercial and regulatory ecosystem for nuclear energy. Governmental support for this transformation is of the essence as a strong sense of direction will be crucial.
CATF’s Report “Nuclear Energy at Scale: A New Pathway to Meet the Climate and Human Development Challenge” outlines a range of commercial and regulatory solutions that would enable nuclear to expand at pace and scale. The solutions outlined below include a more product-based business model, combined with integrated project delivery, to reduce risks, time to market, and costs – including both capital costs as well as risk premiums demanded by lenders – thereby increasing bankability. Regulatory harmonisation and a boost to host country building capability will speed up deployment, whilst de-risked finance will help a product-based industry reach transformative scale.
What is now a vicious circle of poor finance, low scale, and high regulatory risk, can be turned into a virtuous cycle of investor confidence, project certainty, and more efficient regulation. CATF’s analysis suggests that a combination of these measures could result in an overnight cost reduction of as much as 60% from “first-of-a-kind” to “Nth-of-a-kind” reactors. However, strong government commitment and support is crucial for this cost reduction to be realised. The main elements of the solution are:
- Productisation: shift from slow, expensive mega-projects to standardised, manufactured products to reduce costs.
- Large orderbooks: aggregate demand for repeat builds of the same design to achieve cost reductions.
- Plant delivery integration: establish independent nuclear development organisations (INDOs) to streamline project development and deployment.
- Harmonised global licensing: create a Global Licensing Authority (GLA) to provide globally accepted Design Acceptance Certificates (DACs).
- Technical support for new nuclear nations: form an International Technical Support Organisation (ITSO) to assist first-time nuclear countries in overcoming licensing barriers.
- Broader financing access: establish an International Bank of Nuclear Infrastructure (IBNI) to provide financing and support for nuclear programs.
The UK is in a unique position to consider how these solutions could be implemented to the benefit of economy-wide decarbonisation and to lead this transformation internationally.
Small Modular Reactors (SMRs)
SMRs can play a crucial role in the UK’s efforts to manage climate change by providing clean base-load dispatchable electricity and heat. The availability of such ‘clean firm’ power can help to decarbonise industrial systems, mitigate their emissions, and bolster energy security and reliability. There are a number of benefits of SMRs compared to traditional nuclear power, including: lower initial capital investments, potential siting flexibility, promise of serial production in controlled environments, and faster construction timelines in part from reduced on-site labour.
CATF welcomes the UK’s decisive steps towards deployment of SMRs via Great British Nuclear’s SMR competition, which aims to select a technology provider later this year and arrive at Final Investment Decision by 2029.
Harmonisation of licensing and regulation
As the UK embarks on this path it should revisit its approach to licensing new nuclear designs. The current regime necessitates substantial design changes for plants designed overseas – this became apparent in the recent case of Hinkley Point C, where the British version of EDF’s design contains 35% more steel and 25% more concrete than versions of the same plant deployed in other countries.
We would encourage the UK to engage in a collaborative process with other regulators to achieve a high level of design standardisation and minimise future design changes which are likely to hamper technology exports. This can be achieved without surrendering the sovereignty of the UK’s regulatory process, and would enable the UK to lead on SMR deployment internationally, avoiding the significant hurdle that licensing presents. The existing partnership between the UK, Canada and Poland, aiming at deployment of the BWRx design, could act as a test case for such collaboration.
Establecer una licencia por pruebas - "sandbox"
Under the current paradigm, reactor designs are licensed without full-scale testing. Instead, regulators require layers of redundant safety systems and rely on statistical models to determine accident probabilities and safety margins. Data from limited laboratory testing or historic reactor experiments are also utilised. This model contributes to design and licensing complexity, and increases the time and cost of licensing while still leaving some uncertainty as to a reactor’s capabilities.
An alternative approach would be to designate an area, with sufficient oversight, for reactor designers to conduct full-scale testing – ensuring designs behave as predicted under normal and failure conditions – through which designs could achieve full design licenses. This could reduce licensing uncertainty for applicants and investors, particularly for advanced reactors, at the same time as reducing uncertainty for regulators and the public.
Joint Procurement Platform for SMRs
One pivotal step for scale and repeatability involves the establishment of a Joint Procurement Platform for SMRs. This platform, tailored for countries interested in SMRs, would centralise and coordinate demand and connect it with supply. It could be organised around specific regions and coalitions of countries, industry clusters, or public-private consortia, and combine their buying power to commission dozens of units of the same design under umbrella contracts. They could also participate as off-takers through Power Purchase Agreements. This function could be undertaken by Great British Nuclear in the first instance but later opened up to international participation.
This approach would not only enhance economies of scale but also streamline procurement processes, thereby contributing to cost reductions and faster project delivery. Such a unified order would create the necessary scale of demand that can drive the industry into a commoditised product supply chain, by standardising designs, processes and learning through doing. Participating manufacturing supply chains could reap the benefits of producing standardised, commercially available, and “commercial off the shelf (COTS)” SMR components. This will support the creation of regional production for modular designs, and factory-based manufacturing with standardised processes that can lead to standardisation and commoditisation of the industry. This approach is likely not only to decrease costs but also build confidence in the procurement process enabling more effective adoption of this technology internationally.
Fusión
Fusion is an advanced energy source with the potential to produce abundant, zero-emissions power around the world. Paving the way for fusion commercialisation could allow integration of this carbon-free, firm source into the energy mix, and potentially revolutionise how we power the global economy.
The UK has a strong position in this sector, supporting the technology development needed from the public sector as well as creating a friendly environment for private enterprises. This is translated into public support for new fusion projects and the retaining of talent in the sector. Government schemes have had a positive impact on the expansion of fusion technologies, and Government should continue to provide support to facilitate the commercialisation of fusion technology, as well as to progress the development of an appropriate regulatory framework.
Key Policy Recommendations
- Provide funding for fusion energy beyond research, development and demonstration to ensure progress to commercialisation and deployment, including by helping to mitigate risks and uncertainties.
- Continue to support the creation of coalitions and consortiums that allow for collaboration on fusion deployment.
- Support the development of an adequate regulatory framework to facilitate technology development, while making a fair assessment of the potential hazards.
- Collaborate to support the creation of a global fusion industry with a global market, including through the development of harmonised standards and definitions.
Background
What is fusion?
Nuclear fusion occurs when one or more lighter atomic nuclei combine to form a single heavier nucleus, while releasing energy. The process releases energy because the total mass of the resulting single nucleus is less than the mass of the two original nuclei. This reaction takes place in nature: it is the process which powers stars like the Sun.
The fusion reaction refers to the combination of two light nuclei, like a hydrogen nucleus or its isotopes (deuterium and tritium, also written D-T). This combination occurs only when the particles are sufficiently energetic to overcome the Coulomb repulsive force, and for that, a high temperature state called plasma needs to be reached. The D-T reaction has the lowest temperature requirement for ignition. This is by far the most widely studied reaction globally and many fusion concepts are based on it. It will likely be the fusion reaction used for demonstrating the production of grid electricity through fusion, at least in the first phase of commercialisation.
The process to generate a fusion reaction is completely different to the one needed for fission. This is why the technology to be used is also different, with all the subsequent implications in terms of safety and regulation. For most D-T reactions, there will be radiological consequences because neutrons of high energy are produced which will activate the materials of the device, in particular the so-called first wall. Tritium is a radioactive element with a half-life of 12.32 years. Handling and storage of the tritium is therefore an issue of safety concern.
Fusion has been traditionally studied and developed within the public sector, such as national laboratories, universities and other research groups fully funded by taxpayers, meaning there is a strong international collaborative approach to its development.
La energía de fusión tiene el potencial de proporcionar:
- Siempre disponible, energía firme sin residuos radiactivos de alto nivel ni emisiones de gases de efecto invernadero.
- Alto rendimiento energético con una huella de suelo muy pequeña, lo que reduce las barreras de ubicación.
- Accesibilidad en todo el mundo, ya que no depende de recursos naturales regionales.
- Potencial de energía altamente competitivo, produciendo más energía por gramo de combustible que cualquier otro proceso de generación.
Pero los retos persisten:
- Technology advancement: we do not currently have the technology commercialised to tap into this promising energy source. To make fusion a reality, we must advance a diversity of fusion technologies and develop and test fusion plants in real conditions.
- Regulatory certainty: we need clear, specific, and proportionate regulations that provide a reference framework for developers.
- Industry and market cultivation: we must establish a global fusion industry with a global market. The fusion industry is flourishing as new companies and start-ups are being created worldwide, and international collaborations and contracts are evolving quickly.
International collaboration
Sharing UK domestic experience and expertise
Internationally, the UK has long been regarded as a climate leader – it was the first major economy to legislate for net zero and the first to set legally binding carbon budgets, and it has a successful history of climate policy innovation.
The UK has navigated the early stages of the energy transition in a productive manner and has excellent experience to share. It should use this to support others to help meet the global climate challenge. Countries such as Poland could learn from the solutions which have worked well, as well as the potential pitfalls of policy options already explored in the UK, including:
- Support mechanisms for clean energy
- Transitions strategy development, including analytical resources and public involvement
- Climate governance.
Mobilising the full strength of UK diplomacy to ensure global decarbonisation
The UK has an extensive diplomatic network which it has used to foster strong relationships and collaboration on climate with international partners, particularly in the run up to COP26. The UK continues to push for ambitious action in the UNFCCC, and called for the phase out of unabated fossil fuels at COP28. However, recent row backs on domestic climate policy threaten to damage the UK’s international reputation, and have meant the UK is left out of key international coalitions it has historically been a part of, such as the High Ambition Coalition. The UK should use its climate diplomacy to foster decarbonisation across the globe – backed by strong climate action at home – by sharing its experience and learnings, continuing to champion initiatives like the Global Methane Pledge and putting forward concrete steps towards their realisation. The UK should capitalise on its regulatory expertise to lead the way in the development of international standards and their global diffusion, such as its low carbon hydrogen certification criteria.
Supporting emerging and developing economies
Most energy growth and new energy infrastructure development in the future will happen in emerging and developing economies (EMDE). However, EMDE will only embrace clean technologies if they are cheap and enable them to do the same or more than they would do with conventional alternatives. To facilitate clean energy adoption, the UK should support the establishment of clean energy value chains in Africa, coupled with provisions for market access. It should also provide research and development support and partnerships to enable Africa to take the lead in shaping its clean energy future. A CATF study into energy transitions research in Africa found that nearly half of African countries are not covered by current research, only 10% considered development as an outcome of interest, and only 25% of the research papers were produced by researchers based on the continent.
The UK has a strong commitment to supporting developing countries through its International Climate Finance (ICF) and has committed £11.6 billion between 2021-2026. Between April 2011 and March 2022, it is estimated that ICF programmes mobilised £5.7 billion public and £5.2 billion private finance. However, the UK has fallen nearly 40% behind on its £11.6 billion target, and is far from spending its fair share of the $100 billion goal – it is 66% of the way there, with a shortfall of around $2 billion.
However, $100 billion is just “the tip of the iceberg” compared with the trillions of dollars needed annually by 2030 and beyond to transform energy systems, mostly in the growing economies of the developing world. To achieve net-zero emissions globally by mid-century, the IEA reports that we will need at least $4.5 trillion of annual investment in the energy sector – roughly double current investment levels. Gaining access to capital in EMDE is extremely challenging – investment risks such as general country risk, policy uncertainty, governance capacity limits, counterparty payment risks, currency hedging, and historical risk bias have historically limited capital flow from wealthy nations into EMDEs. Because of these risks, the cost capital for such economies can be two to three times higher than similar projects located within wealthy countries, which means otherwise profitable projects are unable to move forward. In the context of rising cost of capital globally, Government support for EMDE is therefore even more crucial; the UK must enhance and continue to deploy international climate finance to support project development and deployment in EMDE.
UK decarbonisation must also be developed as part of a global framework, and the UK should therefore assess its decarbonisation plans not only on the reduction of emissions for the UK, but also on the climate impact in other regions.
Work closely with key partners to achieve decarbonisation goals
As an EU Member State, the UK continuously pushed for ambitious climate action, and supported the development of key climate policies including the Emissions Trading System. Whilst relations between the EU and the UK have remained frosty since the Brexit referendum in 2016, agreement on the UK’s participation in Horizon Europe is a positive step for EU-UK relations. The UK has also been collaborating bilaterally with EU Member States on climate, such as the UK-Germany partnership for cooperation on clean energy. There may be further synergies worth exploring such as the deployment of SMRs between the UK and Poland.
The EU and the UK should look for further opportunities to collaborate on climate and advance clean energy technologies. Collaboration on the transport and storage of CO2, for example, will be particularly important to the EU’s Industrial Carbon Management Strategy. The UK has advanced its CCS policy and project deployment, with the development of business models, CCS clusters and storage resources, and could therefore act as a useful partner going forward. The EU and the UK should also collaborate on standards for low carbon hydrogen to maximise the economic opportunities of trade in hydrogen between the UK and EU Member States.
Nuclear energy relations between the two blocs are currently managed by a separate agreement between Euratom and the UK, focusing on cooperation on safe and peaceful uses of nuclear energy as well as including provisions for research and development. However, as the EU and the UK are both increasingly focused on nuclear energy, a dialogue on licensing and sharing best practices could help to speed up deployment.
The upcoming review of the Trade and Cooperation Agreement should be considered an opportunity to strengthen EU-UK collaboration on climate and energy, and to remove any barriers to successful cooperation, such as regarding the transport and storage of CO2. A joint statement by the European Commission and the UK Government on furthering climate action and clean energy cooperation ahead of COP29 would provide a strong signal globally and help to re-establish the EU and UK’s international leadership on climate following the European and UK elections.
The UK and the US should continue to collaborate on the international stage to support international initiatives and cooperation for climate. Together, they can build international coalitions around key topics such as methane or carbon management, and coordinate to promote global climate ambition and the implementation of international commitments.
Notas a pie de página
- Considering UK gas prices for October 13, 2023 (https://tradingeconomics.com/commodity/uk-natural-gas)
- McKinsey, (2018), Decarbonization of industrial sectors: the next frontier.
- McKinsey, (2020), Net Zero Europe.
- IEA, (2023), Report on Electrification.
- IEA, (2020), Outlook for biogas and Biomethane: Prospects for organic growth.
- IEA, (2023), Report on Innovations.
- Climate Change Committee, (2020), The Sixth Carbon Budget.
- Intergovernmental Panel on Climate Change, (2022), Working Group III’s Contribution to the Sixth Assessment Report, https://www.ipcc.ch/report/sixth-assessment-report-working-group-3/ (IPCC, AR 6)
- University of Cambridge Institute for Sustainability Leadership, (2020), Cement sector deep dive.
- Hutton, (2021), UK steel industry statistics and policy.
- IEA, (2023), CCUS policies and business models.
- CATF, (2023), Unlocking Europe’s CO₂ storage potential.
- Approximately 55% of the ammonia produced worldwide is converted to urea, which takes the form of solid white pellets and is applied as a fertiliser. Urea (CH₄N₂O) contains a carbon atom that is normally sourced from natural gas or coal. It is unclear how the carbon needed to make urea would be economically sourced if the ammonia production process relies entirely on low-carbon (green) hydrogen from water electrolysis without any need for hydrocarbon inputs.
Créditos
Autor del informe
Rebecca Tremain, Head of UK Government Affairs, CATF
Colaboradores principales
Alex Carr, Program Manager, Zero-Carbon Fuels, CATF
Sehila Gonzalez, Global Director, Fusion Energy, CATF
Brandon Locke, Director de Política Europea, Prevención de la Contaminación por Metano, CATF
Toby Lockwood, Technology and Markets Director, Carbon Capture, CATF
Lily Odarno, Director, Energy and Climate Innovation, Africa,CATF
Malwina Qvist, analista principal, CATF
Codie Rossi, Policy Associate, Carbon Capture, CATF
Kasparas Spokas, Director de Perspectivas y Estrategia de Integración, CATF
John Steelman, Deputy Director, Transportation Decarbonization, CATF
James Turrito, Director, Global Campaigns, Methane Pollution Prevention,CATF
Alessia Virone, Government Affairs Director, Europe, CATF
Gus Whakim, Production and Export Director, Zero-Carbon Fuels, CATF