Nuclear Energy: A Clean Firm Power Option for Decarbonising the European Union
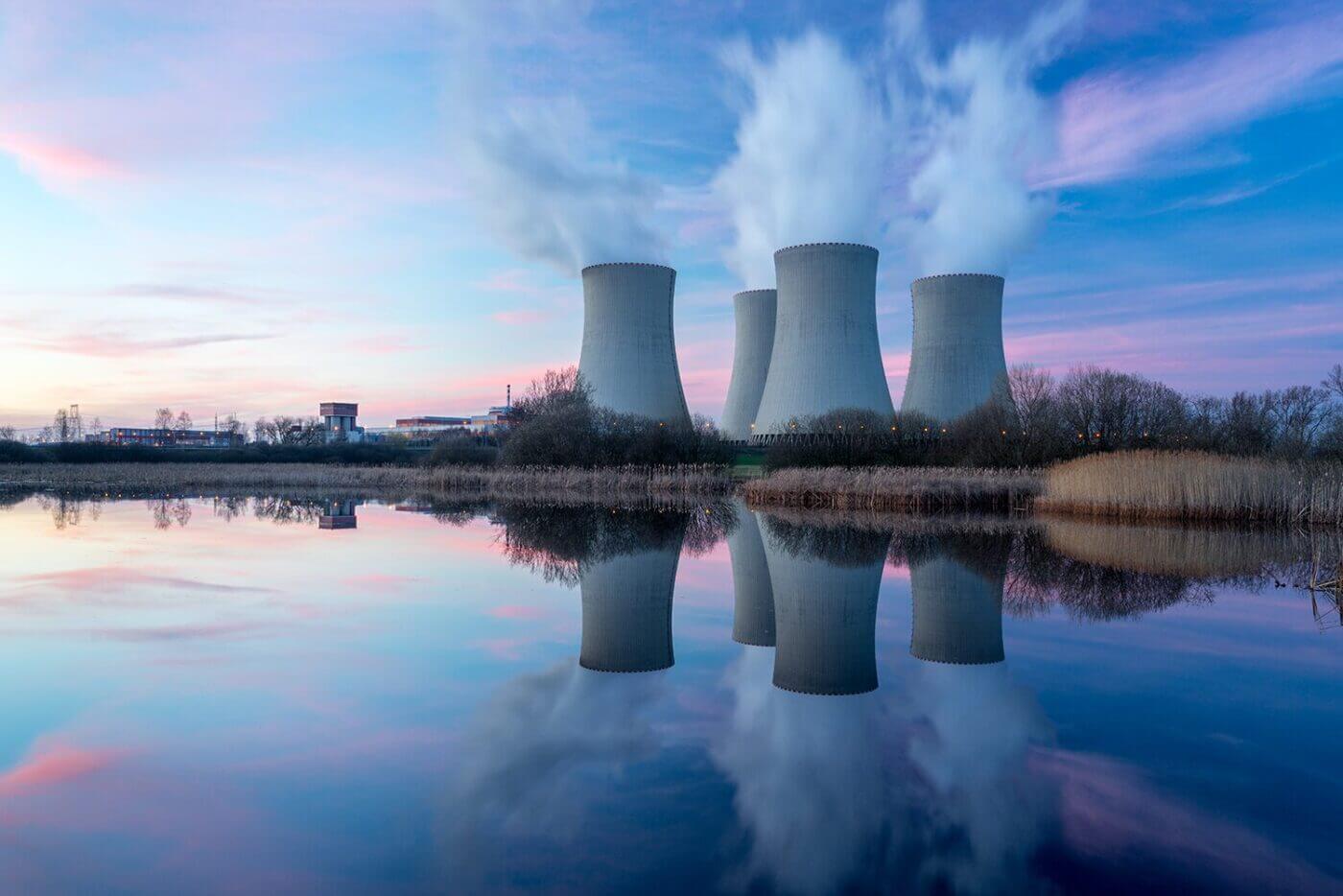
Europe’s electricity grid presents a three-fold challenge:
- The EU must decarbonise its electricity grid in order to address climate change;
- To do this, the EU must increase the speed and scale of electrification, all while balancing affordability and reliability for citizens and industry; and
- At the same time large amount of clean firm power capacity is aging and due to be replaced before 2050.
This is a complex challenge that is further complicated by increasing international industrial competition. Meeting this moment requires a diverse portfolio of solutions. One option, nuclear energy, has gained renewed attention in the European Union, both at the EU and at the Member States levels due to its ability to generate clean firm power.
Figure 1: Electricity production by source, European Union

What is clean firm power, and why consider it?
The world is going to need more energy, not less. Global electricity demand is expected to grow by about one-third to three-quarters by 2050, requiring strategies that limit increasing emissions while meeting this additional demand. Renewable energy sources, such as wind and solar, will be critical globally, but clean firm power offers complementary advantages. Clean firm options like geothermal and nuclear energy can significantly enhance decarbonisation efforts in the EU.
Clean firm power refers to power sources that generate electricity on-demand, regardless of the weather or time of day, with minimal emissions. Clean firm power technologies can achieve very high-capacity factor. Technologies such as nuclear fission, fusion, geothermal (including superhot rock geothermal), combustion with carbon capture and storage, and zero-carbon fuel combustion are considered to be clean firm (this is not an exclusive list). More information is available here.
- Clean firm power reduces the need for overbuilt capacity of wind and solar: A system relying fully on wind and solar energy will have to account for seasonal variations that include many multi-day, even monthly, periods of shortage. At the moment, in the case of shortage, unabated fossil energy is used instead. Clean firm power helps balance these fluctuations without the need for excessive renewable capacity, ultimately lowering the total system cost of the energy transition.
- Clean firm power reduces the need for transmission infrastructure: Renewable energy deployment faces several bottlenecks, including multiyear transmission connection queues and permitting delays. More energy dense clean firm power, which can be more geographically flexible, reduces the reliance on expansive new transmission infrastructure required to connect distant renewable projects. This is crucial in Europe, where limited land availability and increasing local opposition make siting new renewable projects close to transmission lines increasingly difficult.
- Clean firm power reduces the need for fossil fuel back-up generation: To address renewable variability, the current EU energy system often relies on unabated fossil fuels as backup. Clean firm power, such as advanced nuclear and geothermal can replace fossil fuel backups, ensuring reliable electricity even during periods of low wind and solar generation. This is vital for meeting decarbonisation targets without falling back on carbon-intensive solutions.
- Clean firm power can enhance the speed of decarbonisation: Potential delays in renewable energy deployment—due to transmission connection issues and slow permitting processes—jeopardise timely decarbonisation efforts. Clean firm power can provide a more immediate, scalable solution to ensure that energy systems can decarbonise without waiting for long-term renewable projects and long-duration energy storage or clean hydrogen supplies that would be better prioritised elsewhere. This enables faster and more secure progress toward climate goals.
Value and role of clean firm power technologies for deep decarbonisation
The predominant approach driving the deployment of weather-dependent renewable technologies exposes Member States to potentially higher overall energy transition costs. As renewable deployment lags due to transmission connection queues and other limitations, the risk increases that countries may fail to meet decarbonisation targets in time.
One significant challenge with conventional renewable energy is seasonal fluctuation. A system relying fully on wind and solar would need to account for multi-day, even monthly, periods of energy shortfall (See Figure 2). Moreover, variations in solar and wind output are often correlated, compounding potential shortfalls. Increased interconnection and energy storage are often proposed as solutions to daily fluctuations. While these can improve the use of renewable resources, they are unlikely to fully resolve the issue on multiday timescale.
Figure 2: Temporal variability of solar and wind resources and electricity demand in Germany
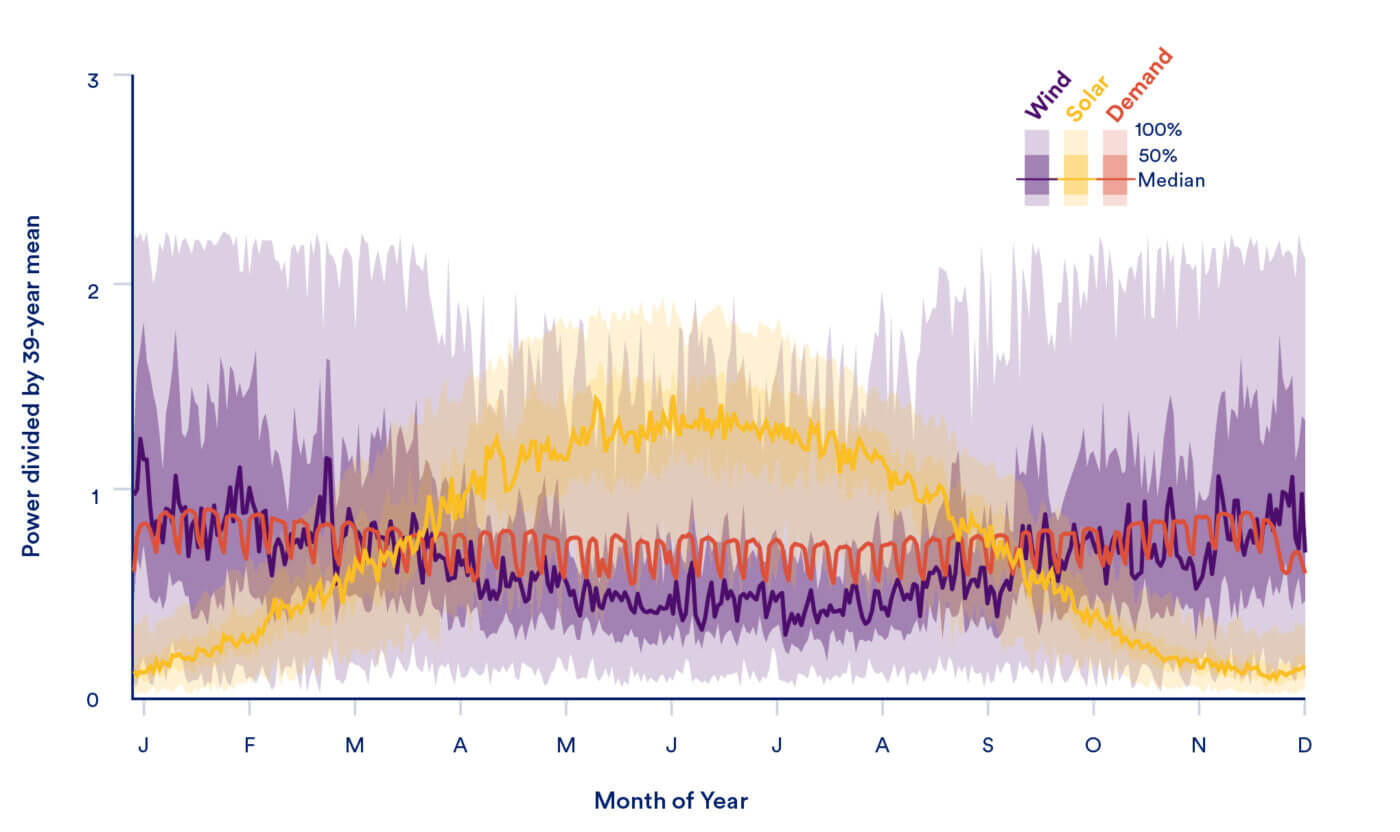
To address this challenge, the EU energy system currently falls back on unabated fossil fuels, which drives up greenhouse gas emissions. Overbuilding wind and solar generators is another option, but it would be costly and face serious land-use constraints (See Figure 3). Long-duration energy storage is often mentioned as a potential solution, but such systems are not yet available at the necessary scale or cost1 for short- or medium-term deployment. Similarly, while clean hydrogen is technically suitable, it is likely to be a scarce resource that should be reserved for sectors where no viable alternatives exist.
Figure 3: Land-use considerations for development of electricity generation infrastructure
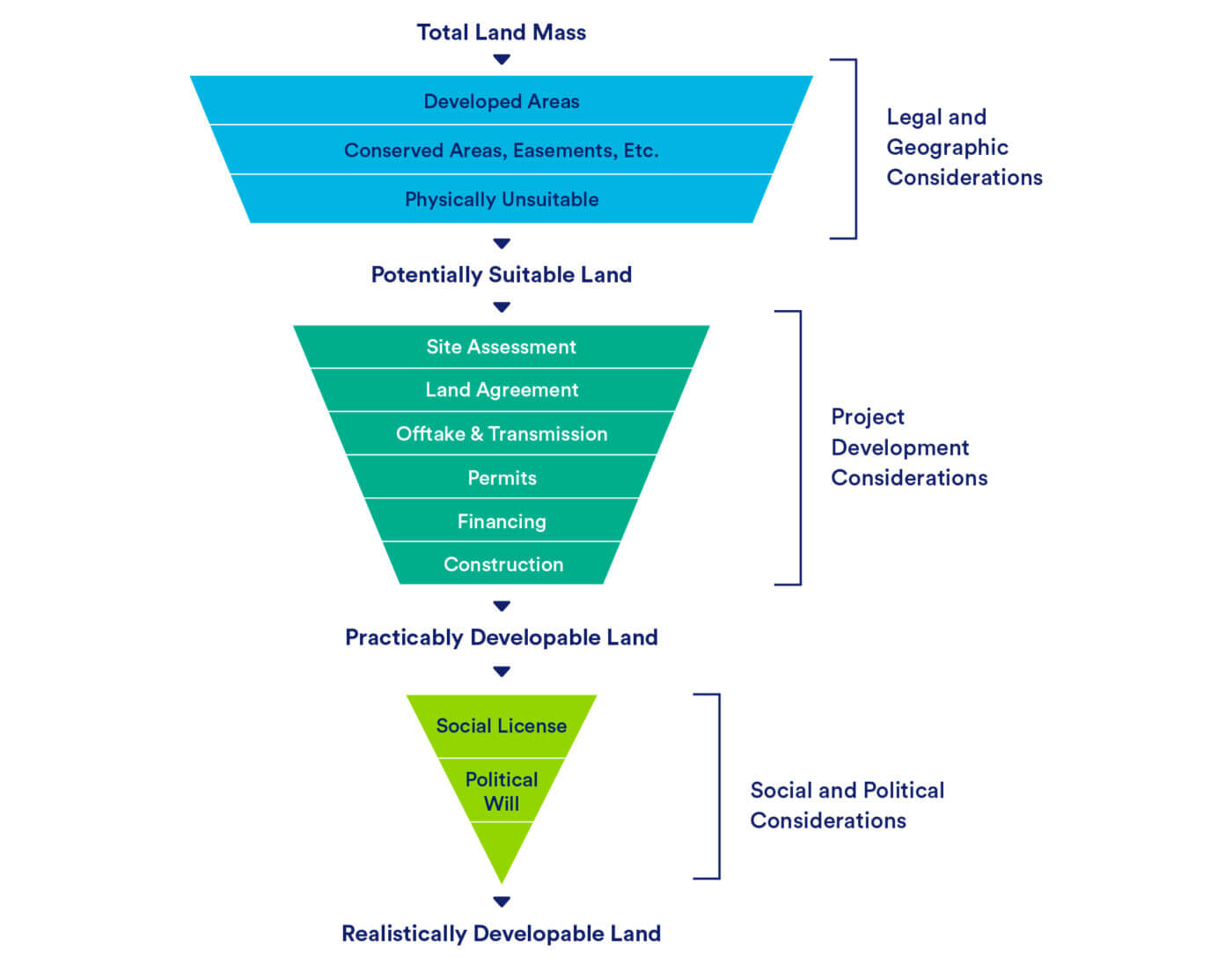
Renewable generators like wind and solar also raise questions about land use. The EU has limited available land with many competing uses. Member States must consider the space required, along with its costs and climate impacts, to produce the same quantity of energy. Furthermore, as shown in figure 3 not all technically suitable locations for clean energy development are practically or politically feasible. Appropriate sites are increasingly difficult to find due to rising local opposition and greater distances from existing transmission lines. In this critical time, renewable deployment should be accelerating, yet grid connection queues and permitting delays threaten the achievement of climate targets. As a result, while wind and solar must be developed at an unprecedented scale and rate, complementary energy sources will be required to balance risks and limitations, and to hedge the EU’s transition strategy.
Figure 4: Relative magnitude of surface area used for different energy sources to meet the total energy demand of Italy
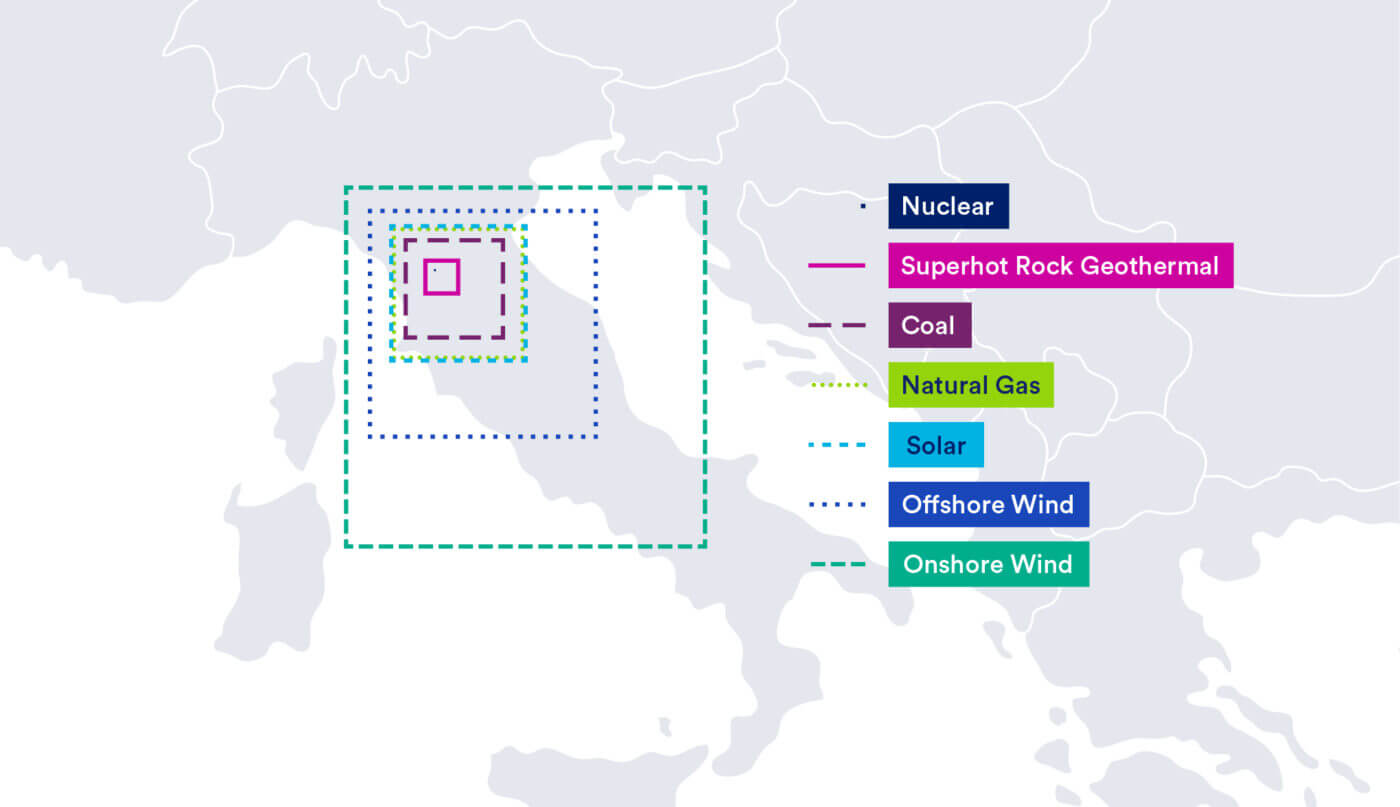
For the EU to achieve a fully decarbonised energy system with reliable, affordable, and sustainable energy, multiple energy sources will be necessary. This is where clean firm power can play a vital role. Clean firm power can help address seasonal reliability at a lower cost, offering generation that is always available and largely independent of the weather fluctuations. Europe should focus on at least two promising clean firm options: geothermal and nuclear energy and develop supporting policies that will accelerate the deployment of those solutions to meet its climate targets.
What role can the EU play in nuclear energy deployment?
While the EU should not dictate the makeup of its Member State’s energy systems, it can play a critical role in coordinating the deployment of nuclear energy within the bloc by providing enabling structures to accelerate nuclear energy deployment with high contribution from the EU across the whole value chain. Key actions the EU should take include:
Developing an EU strategy for small modular reactors (SMRs)
Although several Member States have announced intentions to develop and deploy SMRs, significant challenges remain that could hinder the progress of such projects in Europe.
To address these challenges, the EU should create a comprehensive strategy for the development and deployment of SMRs, as requested by the European Parliament in December 2023. This strategy should build on the work of the EU SMR Industrial Alliance, addressing the challenges and solutions to SMR deployment in Europe. Key areas to focus on include licensing harmonisation, design standardisation, public acceptance, identification of the most promising designs, development of a robust supply chain, investment challenges, research and development needs, human resources, and spent fuel management strategies.
Coordinating technology choices leading to pan-European orderbooks
The nuclear energy offering currently boasts over 80 designs in development, a vast majority of which are so called ‘paper reactors’ and underdeveloped but innovative designs with few committed prospects. This market will need to undergo a period of consolidation leading to a smaller number of most attractive designs progressing to development. This would lead to more efficient allocation of development funds and resulting in accelerated delivery timeline.
Nuclear energy technologies need to come down the cost curve (similarly to what solar and wind technologies have achieved in the last decade or two). This can be best achieved by repeat build of the same standardised design which will lead to learning by doing in construction and boost supply chains development. It is clear that first-of-a-kind (FOAK) units will come with a high price tag, as did early solar and wind projects in the early 2000’s, however, subsequent builds will drive the cost reduction leading to Nth-of-a-kind (NOAK) costs after approximately 5-10 initial units.
A regional orderbook of reactors, coordinated by the EU, is likely to drive investment into regional supply chains, increasing economic growth and boosting Europe’s contribution to new build plants. This benefit is unlikely to materialise if we have a high level of variety in technology choices, as they would lead to fragmented supply chains and less unit-to-unit learning. With a regional orderbook coordinated at the EU level, Member States could align in the early stage of their SMRs plans, and decide together to pursue a small subset of nuclear technologies, leading to a low number of designs being developed and the cost-benefits it would bring.
Establishing a joint procurement platform for new build nuclear
A key step in scaling and replicating nuclear projects, especially SMRs, would be the creation of a Joint Procurement Platform. This platform would centralise and coordinate procurement efforts among Member States developing new build nuclear energy, connecting it with supply, and facilitating the delivery of the projects. It could be organised around specific regions, coalitions of countries, industry clusters, or public-private consortia, leveraging collective buying power to commission multiple units of reactors, plant components, or civil works under umbrella contracts. Such an approach would increase the purchasing power of the buyers but also improve the regional and local supply chain signals driving the economies of numbers, turning SMRs into a commoditised product through design standardisation, streamlined processes, and the benefits of “learning by doing.”
Conclusion
Nuclear energy is a clean firm power source that can play a valuable role to achieving a fully decarbonised, reliable, affordable, and secure energy system in the EU. By complimenting the limitations of weather-dependent renewables, clean firm power provides the foundation for a resilient energy transition, enabling the bloc to meet its climate targets without compromising energy security or cost-efficiency.
Frequently Asked Questions
1. Why consider nuclear energy, and how does it work?
Nuclear energy is a carbon-free, energy-dense technology capable of producing low-carbon energy (electricity and heat) from a small land footprint, making it a potentially useful clean firm complement to renewables or a useful carbon-free energy alternative on its own. While it can be deployed in many locations, sites near large bodies of water are preferable for optimal economics.
Nuclear energy generation works by using the heat produced from nuclear fission reactions in uranium fuel (or other fissile material) to create steam. This steam then drives a turbine- generator set to produce electricity or is used directly for industrial applications. Most of the current global nuclear fleet consists of generation II and III light water reactors (LWRs). More modern technology that is currently being deployed can be classified as generation III/III+ capable of increased performance, flexibility, and safety compared to its predecessor. Other nuclear energy technologies include:
- Small Modular Reactors: Small Modular Reactors (SMRs) are often defined as LWRs under 300MWe. They suit smaller grids and industrial applications and could also benefit from factory construction of units, easier access to financing due to smaller absolute investment size, and the ability to add more units as demand grows. SMRs are being developed in 18 countries, with the EU targeting successful deployment of its first SMRs by the early 2030s.
- Advanced Reactor Technologies: Advanced reactor (AR) technologies typically refer to Generation IV technologies that use novel types of nuclear fuel and coolants other than water to deliver significantly improved attributes such as passive safety, operational flexibility, and long operation periods between refuelling cycles. These technologies are still in the development and demonstration phase, and while they are currently pre-commercial, they have the potential to contribute to climate goals in the future.
The interactive map below shows locations and details of projects underway globally. These details can also be viewed in this chart.
2. Is nuclear energy safe?
Nuclear energy technologies are subject to intense regulation and high levels of scrutiny by national regulatory authorities and international organisations, such as the International Atomic Energy Agency (IAEA). The nuclear industry has experienced three major accidents: Three Mile Island, Chernobyl, and Fukushima. These accidents occurred within 20,000 reactor-years of operational experience worldwide, and therefore, nuclear power is classified as one of the safest power generation technologies in the world (93 times safer than natural gas, 153 times safer than biomass, and 820 times safer than coal – based on data from ‘Our World in Data’’). Conventional reactors being built today, as well as more advanced designs, typically also include passive safety features. This means their safety systems are not dependent on additional external power or operators’ actions but rather on natural phenomena such as gravity to shut down reactors safely in case of an issue.
3. What about nuclear waste?
Nuclear waste is a common environmental concern in the scale-up of nuclear energy. Currently, spent nuclear fuel is mostly stored at nuclear power plants. Initially, it is kept in spent fuel pools near reactors for about five years, where water cools the fuel and removes decay heat. After this period, spent fuel is transferred to dry cask storage, where air cooling suffices. This storage requires minimal space and is typically located within the plant perimeter.
The final step is either reprocessing to recycle materials or disposal, with disposal more often pursued due to proliferation concerns. Although spent fuel disposal is seen as urgent and unsolved, effective technical solutions already exist. For example, Finland’s Onkalo facility serves as a successful example of consent-based siting which offers indefinite storage for high-level nuclear waste. Governments should implement pathways for final disposal, focusing on considerate and transparent processes that make use of consent-based siting to identify potential storage sites worldwide. In the meantime, dry cask storage remains a safe, sustainable option as final solutions develop.
4. What does nuclear cost?
Nuclear energy has a high upfront construction cost (CAPEX), and relatively low operational (OPEX) and decommissioning costs. CAPEX for recent builds notably ranges from $4000 per kilowatt of installed capacity in the UAE to $12000 per kilowatt in the USA. These costs escalated in recent decades, especially in the western world, as projects struggled with number of difficulties and were completed at multiple of the original schedule and budget. Majority of those difficulties can be ascribed to loss of nuclear know-how after a multidecade break in nuclear construction resulting in atrophy of the industrial capabilities. However, as supply chains and industry regain competence costs are expected to decrease. This can be already seen in recent builds in UEA and USA where learning between consecutive units allowed for circa 30% cost reduction.
1 Long term duration storage is currently at an order of magnitude cost higher than it needs to be to become a cost effective solution to this issue.